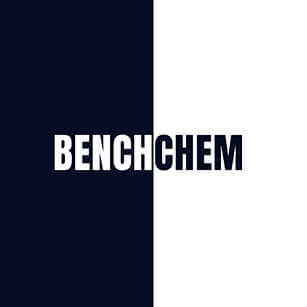
POLY(3-DODECYLTHIOPHENE-2 5-DIYL)
- Click on QUICK INQUIRY to receive a quote from our team of experts.
- With the quality product at a COMPETITIVE price, you can focus more on your research.
Overview
Description
Poly(3-dodecylthiophene-2,5-diyl): is a conducting polymer belonging to the family of poly(3-alkylthiophenes). It is known for its high conductivity and reversible electrochemistry. This compound is characterized by its regioregular structure, which contributes to its excellent electrical properties. It forms a shiny red-purple film and is used in various electronic applications .
Mechanism of Action
- P3DDT primarily interacts with electronic components in organic electronic devices. Its primary targets include interfaces within thin films, such as those in organic solar cells, organic light-emitting diodes (OLEDs), and thin-film transistors (TFTs) .
- Environmental factors influence P3DDT’s efficacy and stability:
Target of Action
Action Environment
Biochemical Analysis
Biochemical Properties
POLY(3-DODECYLTHIOPHENE-2,5-DIYL) plays a significant role in biochemical reactions due to its conductive nature. It interacts with various biomolecules, including enzymes and proteins, through electrostatic and hydrophobic interactions . These interactions can influence the activity of enzymes and the stability of protein structures. For example, POLY(3-DODECYLTHIOPHENE-2,5-DIYL) can interact with cytochrome P450 enzymes, affecting their electron transfer processes .
Cellular Effects
POLY(3-DODECYLTHIOPHENE-2,5-DIYL) has been shown to influence cellular processes by modulating cell signaling pathways and gene expression . It can affect the function of various cell types, including neuronal and epithelial cells, by altering their membrane potentials and ion channel activities . Additionally, POLY(3-DODECYLTHIOPHENE-2,5-DIYL) can impact cellular metabolism by influencing the activity of metabolic enzymes and the production of reactive oxygen species .
Molecular Mechanism
At the molecular level, POLY(3-DODECYLTHIOPHENE-2,5-DIYL) exerts its effects through binding interactions with biomolecules and modulation of enzyme activities . It can inhibit or activate enzymes by altering their conformations and electron transfer properties . Furthermore, POLY(3-DODECYLTHIOPHENE-2,5-DIYL) can influence gene expression by interacting with transcription factors and other regulatory proteins .
Temporal Effects in Laboratory Settings
In laboratory settings, the effects of POLY(3-DODECYLTHIOPHENE-2,5-DIYL) can change over time due to its stability and degradation properties . The polymer is relatively stable under ambient conditions but can degrade under extreme temperatures or oxidative environments . Long-term exposure to POLY(3-DODECYLTHIOPHENE-2,5-DIYL) can lead to changes in cellular function, including alterations in cell proliferation and apoptosis rates .
Dosage Effects in Animal Models
The effects of POLY(3-DODECYLTHIOPHENE-2,5-DIYL) vary with different dosages in animal models . At low doses, the polymer may enhance cellular functions and promote tissue regeneration . At high doses, POLY(3-DODECYLTHIOPHENE-2,5-DIYL) can exhibit toxic effects, including oxidative stress and inflammation . Threshold effects have been observed, where the polymer’s impact on cellular functions changes significantly at specific concentration ranges .
Metabolic Pathways
POLY(3-DODECYLTHIOPHENE-2,5-DIYL) is involved in various metabolic pathways, interacting with enzymes and cofactors that regulate metabolic flux . It can affect the levels of metabolites by modulating the activity of key metabolic enzymes . For instance, POLY(3-DODECYLTHIOPHENE-2,5-DIYL) can influence the glycolytic pathway by interacting with hexokinase and phosphofructokinase .
Transport and Distribution
Within cells and tissues, POLY(3-DODECYLTHIOPHENE-2,5-DIYL) is transported and distributed through interactions with transporters and binding proteins . It can accumulate in specific cellular compartments, such as the cytoplasm and mitochondria, affecting their functions . The polymer’s distribution is influenced by its molecular weight and hydrophobicity .
Subcellular Localization
POLY(3-DODECYLTHIOPHENE-2,5-DIYL) exhibits specific subcellular localization patterns, which can affect its activity and function . It can be directed to particular organelles, such as the endoplasmic reticulum and lysosomes, through targeting signals and post-translational modifications . This localization is crucial for the polymer’s role in cellular processes and its interactions with biomolecules .
Preparation Methods
Synthetic Routes and Reaction Conditions: Poly(3-dodecylthiophene-2,5-diyl) can be synthesized through oxidative coupling polymerization. This method involves the polymerization of 3-dodecylthiophene monomers using an oxidizing agent such as ferric chloride (FeCl3) in an organic solvent like chloroform .
Industrial Production Methods: In industrial settings, the production of poly(3-dodecylthiophene-2,5-diyl) involves similar oxidative polymerization techniques but on a larger scale. The reaction conditions are optimized to ensure high yield and purity of the polymer. The polymer is then purified and processed into the desired form for various applications .
Chemical Reactions Analysis
Types of Reactions: Poly(3-dodecylthiophene-2,5-diyl) undergoes several types of chemical reactions, including:
Oxidation: The polymer can be oxidized to form a doped state, which enhances its conductivity.
Reduction: Reduction reactions can revert the polymer to its neutral state.
Substitution: The polymer can undergo substitution reactions where functional groups are introduced to modify its properties.
Common Reagents and Conditions:
Oxidation: Ferric chloride (FeCl3) in chloroform.
Reduction: Sodium borohydride (NaBH4) in methanol.
Substitution: Various alkyl halides in the presence of a base.
Major Products Formed:
Oxidation: Doped poly(3-dodecylthiophene-2,5-diyl) with enhanced conductivity.
Reduction: Neutral poly(3-dodecylthiophene-2,5-diyl).
Substitution: Functionalized poly(3-dodecylthiophene-2,5-diyl) with modified properties.
Scientific Research Applications
Poly(3-dodecylthiophene-2,5-diyl) has a wide range of scientific research applications, including:
Chemistry: Used in the development of organic electronics, such as organic solar cells and field-effect transistors.
Biology: Employed in biosensors for detecting biological molecules.
Medicine: Investigated for use in drug delivery systems due to its biocompatibility.
Comparison with Similar Compounds
Poly(3-dodecylthiophene-2,5-diyl) can be compared with other similar compounds such as:
Poly(3-hexylthiophene-2,5-diyl): Known for its high mobility and used in organic photovoltaics.
Poly(3-octylthiophene-2,5-diyl): Similar to poly(3-dodecylthiophene-2,5-diyl) but with different alkyl side chains, affecting its solubility and electronic properties.
Poly(3-decylthiophene-2,5-diyl): Another member of the poly(3-alkylthiophene) family with distinct properties due to its alkyl side chain length.
Poly(3-dodecylthiophene-2,5-diyl) is unique due to its specific alkyl side chain length, which provides a balance between solubility and electronic properties, making it suitable for a wide range of applications .
Biological Activity
Poly(3-dodecylthiophene-2,5-diyl) (P3DDT) is a conjugated polymer that has gained attention for its unique properties and potential applications in various fields, particularly in biology and medicine. This article explores the biological activity of P3DDT, including its biochemical interactions, cellular effects, and implications in drug delivery and biosensing.
P3DDT exhibits significant biochemical properties due to its conductive nature. It interacts with various biomolecules through electrostatic and hydrophobic interactions , which can influence enzyme activity and protein stability. These interactions are crucial for its application in biosensors and drug delivery systems .
Table 1: Interaction Mechanisms of P3DDT
Interaction Type | Description |
---|---|
Electrostatic | Attraction or repulsion between charged groups on P3DDT and biomolecules. |
Hydrophobic | Non-polar interactions that stabilize protein structures when P3DDT is present. |
2. Cellular Effects
P3DDT has been shown to modulate key cellular processes, influencing cell signaling pathways and gene expression . Research indicates that P3DDT can enhance or inhibit specific signaling pathways depending on its concentration and the cellular context.
Case Study: Gene Expression Modulation
In a study involving human cell lines, P3DDT was found to upregulate the expression of genes involved in antioxidant responses while downregulating pro-inflammatory cytokines. This suggests potential therapeutic applications in inflammatory diseases.
The molecular mechanism by which P3DDT exerts its biological effects involves binding interactions with various biomolecules, leading to the modulation of enzyme activities. For instance, P3DDT can act as a co-factor for certain enzymes, enhancing their catalytic efficiency.
4. Dosage Effects in Animal Models
The biological activity of P3DDT varies significantly with dosage in animal models. Studies have shown that low doses can promote cell proliferation, while higher doses may induce cytotoxic effects. This duality highlights the importance of dosage optimization for therapeutic applications .
Table 2: Dosage Effects Observed in Animal Studies
Dosage (mg/kg) | Effect on Cell Proliferation | Cytotoxicity |
---|---|---|
1 | Increased | None |
10 | Moderate increase | Mild |
50 | Decreased | High |
5. Metabolic Pathways
P3DDT is involved in several metabolic pathways within cells. Its interactions with enzymes can alter metabolic fluxes, impacting energy production and biosynthesis processes. Specifically, it has been shown to influence pathways related to lipid metabolism and oxidative stress responses .
6. Transport and Distribution
The transport and distribution of P3DDT within biological systems are facilitated by its interactions with transport proteins and cellular membranes. Studies indicate that P3DDT can enhance the permeability of cell membranes, aiding in the delivery of therapeutic agents .
7. Subcellular Localization
P3DDT exhibits specific localization patterns within cells, which can affect its biological activity. For example, when localized to mitochondria, it may enhance mitochondrial function and reduce oxidative stress .
8. Research Applications
The unique properties of P3DDT make it suitable for various research applications:
- Biosensors : Utilized for detecting biomolecules due to its conductive properties.
- Drug Delivery Systems : Investigated for its ability to encapsulate drugs and facilitate targeted delivery.
- Organic Electronics : Employed in the development of organic solar cells and field-effect transistors due to its electronic properties .
Properties
CAS No. |
137191-59-4 |
---|---|
Molecular Formula |
C8H8BrIO2 |
Molecular Weight |
0 |
Origin of Product |
United States |
Q1: How does the side chain length of POLY(3-ALKYLTHIOPHENE-2,5-DIYL)S (P3ATs) impact their backbone dynamics and what implications does this have for their application in devices like OTFTs?
A1: Research suggests that longer side chains in P3ATs, like the dodecyl chains in P3DDT, lead to enhanced backbone motion compared to shorter chain variants like POLY(3-HEXYLTHIOPHENE-2,5-DIYL) (P3HT) . This increased backbone flexibility, confirmed through molecular dynamics simulations and experimental techniques like quasi-elastic neutron scattering (QENS) and Nuclear Magnetic Resonance (NMR), can influence charge transport properties crucial for the performance of organic thin film transistors (OTFTs) . While P3DDT's faster backbone dynamics could potentially facilitate charge carrier mobility, further research is needed to fully understand its impact on OTFT performance parameters like mobility, threshold voltage, and on/off ratio.
Q2: What experimental techniques provide insights into the structural characteristics of P3DDT?
A2: Several experimental techniques provide valuable insights into the structural features of P3DDT. Small-angle neutron scattering (SANS) revealed that regiorandom P3DDT exhibits an amorphous structure at around 300 K, although a microphase separation between the side chains and backbones was observed . This information is crucial for understanding the arrangement of P3DDT chains and how they influence the material's overall properties. Additionally, NMR spectroscopy, specifically spin-lattice relaxation (T1) measurements, corroborated the faster motion of side chain atoms situated farther from the backbone , supporting the findings from molecular dynamics simulations.
Disclaimer and Information on In-Vitro Research Products
Please be aware that all articles and product information presented on BenchChem are intended solely for informational purposes. The products available for purchase on BenchChem are specifically designed for in-vitro studies, which are conducted outside of living organisms. In-vitro studies, derived from the Latin term "in glass," involve experiments performed in controlled laboratory settings using cells or tissues. It is important to note that these products are not categorized as medicines or drugs, and they have not received approval from the FDA for the prevention, treatment, or cure of any medical condition, ailment, or disease. We must emphasize that any form of bodily introduction of these products into humans or animals is strictly prohibited by law. It is essential to adhere to these guidelines to ensure compliance with legal and ethical standards in research and experimentation.