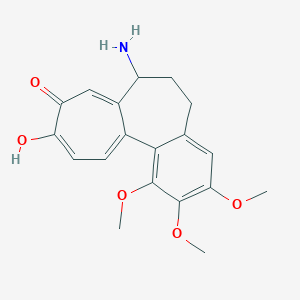
Deacetylcolchiceine
Overview
Description
Deacetylcolchiceine is a derivative of colchicine, an alkaloid found in plants such as Colchicum autumnale and Gloriosa superba. Colchicine and its derivatives, including this compound, are known for their cytotoxic properties and have been used in various medicinal applications, particularly in the treatment of gout and familial Mediterranean fever .
Mechanism of Action
Target of Action
The primary target of Deacetylcolchiceine is tubulin , a globular protein that is the main constituent of microtubules in cells . Tubulin plays a crucial role in maintaining cell structure, cell division, and intracellular transport .
Mode of Action
This compound interacts with tubulin by binding to it and disrupting microtubule action . This prevents the polymerization of tubulin, thereby inhibiting the formation of microtubules . Additionally, this compound has been shown to stimulate the intrinsic GTPase activity of tubulin . This interaction induces apoptosis and activates the JNK/SAPK signals .
Biochemical Pathways
The interaction of this compound with tubulin affects the microtubule dynamics, which is a crucial part of many cellular processes, including cell division and intracellular transport . By disrupting microtubule action, this compound can affect these processes and their downstream effects .
Result of Action
The binding of this compound to tubulin and the subsequent disruption of microtubule action can lead to cell cycle arrest and apoptosis . This can have significant effects at the molecular and cellular levels, potentially making this compound a valuable tool in the treatment of diseases characterized by uncontrolled cell proliferation .
Biochemical Analysis
Biochemical Properties
Deacetylcolchiceine interacts with several biomolecules, most notably tubulin . Tubulin is a key protein involved in the formation of microtubules, which are essential for cell division and structure . By binding to tubulin, this compound prevents the formation of these microtubules, disrupting normal cell function .
Cellular Effects
The disruption of microtubule formation by this compound has profound effects on cellular processes. It can inhibit cell division, alter cell signaling pathways, and affect gene expression . These effects can influence various types of cells and cellular processes, including cell growth and differentiation .
Molecular Mechanism
This compound exerts its effects at the molecular level primarily through its interaction with tubulin . By binding to tubulin, it prevents the polymerization of tubulin into microtubules . This disruption can lead to changes in gene expression and cellular signaling, as many of these processes rely on the normal function of microtubules .
Dosage Effects in Animal Models
The effects of this compound in animal models can vary based on the dosage. High doses of similar compounds like colchicine have been shown to have toxic effects . Specific dosage effects of this compound in animal models have not been extensively studied.
Metabolic Pathways
It is known that colchicine, a related compound, is metabolized in the liver
Transport and Distribution
It is known that similar compounds like colchicine can be transported across cell membranes and distributed throughout the body .
Subcellular Localization
Given its interaction with tubulin, it is likely that it localizes to areas of the cell where microtubules are found
Preparation Methods
Synthetic Routes and Reaction Conditions: Deacetylcolchiceine can be synthesized from colchicine through a series of chemical reactions. One common method involves the methylation of colchicine using diazomethane, followed by treatment with trifluoroacetylglycolyl chloride in pyridine. This process yields a mixture of deacetylcolchicine and deacetylisocolchicine, which can be separated by chromatography .
Industrial Production Methods: Industrial production of this compound typically follows similar synthetic routes as laboratory methods but on a larger scale. The process involves careful control of reaction conditions to ensure high yield and purity of the final product. Chromatographic techniques are often employed to purify the compound .
Chemical Reactions Analysis
Types of Reactions: Deacetylcolchiceine undergoes various chemical reactions, including:
Oxidation: The compound can be oxidized to form different derivatives.
Reduction: Reduction reactions can modify the functional groups present in this compound.
Substitution: Substitution reactions can introduce new functional groups into the molecule.
Common Reagents and Conditions:
Oxidation: Common oxidizing agents include potassium permanganate and chromium trioxide.
Reduction: Reducing agents such as sodium borohydride and lithium aluminum hydride are often used.
Substitution: Reagents like trifluoroacetylglycolyl chloride in pyridine are used for substitution reactions.
Major Products: The major products formed from these reactions include colchifoline and isocolchifoline, which are derivatives of this compound .
Scientific Research Applications
Deacetylcolchiceine has a wide range of scientific research applications:
Chemistry: It is used as a precursor in the synthesis of various colchicine derivatives.
Biology: The compound is studied for its effects on microtubule dynamics and cell division.
Medicine: this compound and its derivatives are investigated for their potential in treating cancer, gout, and other inflammatory conditions.
Industry: The compound is used in the development of pharmaceuticals and as a research tool in drug discovery
Comparison with Similar Compounds
Colchicine: The parent compound from which deacetylcolchiceine is derived.
Demecolcine: A derivative of colchicine with similar cytotoxic properties.
Colchifoline: A derivative formed from this compound through specific chemical reactions.
Uniqueness: this compound is unique in its specific binding affinity to tubulin and its ability to disrupt microtubule dynamics. This makes it a valuable compound in both research and therapeutic applications, particularly in the study of cell division and the development of anti-cancer drugs .
Properties
IUPAC Name |
7-amino-10-hydroxy-1,2,3-trimethoxy-6,7-dihydro-5H-benzo[a]heptalen-9-one | |
---|---|---|
Source | PubChem | |
URL | https://pubchem.ncbi.nlm.nih.gov | |
Description | Data deposited in or computed by PubChem | |
InChI |
InChI=1S/C19H21NO5/c1-23-16-8-10-4-6-13(20)12-9-15(22)14(21)7-5-11(12)17(10)19(25-3)18(16)24-2/h5,7-9,13H,4,6,20H2,1-3H3,(H,21,22) | |
Source | PubChem | |
URL | https://pubchem.ncbi.nlm.nih.gov | |
Description | Data deposited in or computed by PubChem | |
InChI Key |
IRVWPZRYDQROLU-UHFFFAOYSA-N | |
Source | PubChem | |
URL | https://pubchem.ncbi.nlm.nih.gov | |
Description | Data deposited in or computed by PubChem | |
Canonical SMILES |
COC1=C(C(=C2C(=C1)CCC(C3=CC(=O)C(=CC=C32)O)N)OC)OC | |
Source | PubChem | |
URL | https://pubchem.ncbi.nlm.nih.gov | |
Description | Data deposited in or computed by PubChem | |
Molecular Formula |
C19H21NO5 | |
Source | PubChem | |
URL | https://pubchem.ncbi.nlm.nih.gov | |
Description | Data deposited in or computed by PubChem | |
DSSTOX Substance ID |
DTXSID90875143 | |
Record name | TRIMETHYLCOLCHICINIC ACID | |
Source | EPA DSSTox | |
URL | https://comptox.epa.gov/dashboard/DTXSID90875143 | |
Description | DSSTox provides a high quality public chemistry resource for supporting improved predictive toxicology. | |
Molecular Weight |
343.4 g/mol | |
Source | PubChem | |
URL | https://pubchem.ncbi.nlm.nih.gov | |
Description | Data deposited in or computed by PubChem | |
CAS No. |
3482-37-9 | |
Record name | Deacetylcolchiceine | |
Source | DTP/NCI | |
URL | https://dtp.cancer.gov/dtpstandard/servlet/dwindex?searchtype=NSC&outputformat=html&searchlist=36796 | |
Description | The NCI Development Therapeutics Program (DTP) provides services and resources to the academic and private-sector research communities worldwide to facilitate the discovery and development of new cancer therapeutic agents. | |
Explanation | Unless otherwise indicated, all text within NCI products is free of copyright and may be reused without our permission. Credit the National Cancer Institute as the source. | |
Retrosynthesis Analysis
AI-Powered Synthesis Planning: Our tool employs the Template_relevance Pistachio, Template_relevance Bkms_metabolic, Template_relevance Pistachio_ringbreaker, Template_relevance Reaxys, Template_relevance Reaxys_biocatalysis model, leveraging a vast database of chemical reactions to predict feasible synthetic routes.
One-Step Synthesis Focus: Specifically designed for one-step synthesis, it provides concise and direct routes for your target compounds, streamlining the synthesis process.
Accurate Predictions: Utilizing the extensive PISTACHIO, BKMS_METABOLIC, PISTACHIO_RINGBREAKER, REAXYS, REAXYS_BIOCATALYSIS database, our tool offers high-accuracy predictions, reflecting the latest in chemical research and data.
Strategy Settings
Precursor scoring | Relevance Heuristic |
---|---|
Min. plausibility | 0.01 |
Model | Template_relevance |
Template Set | Pistachio/Bkms_metabolic/Pistachio_ringbreaker/Reaxys/Reaxys_biocatalysis |
Top-N result to add to graph | 6 |
Feasible Synthetic Routes
Disclaimer and Information on In-Vitro Research Products
Please be aware that all articles and product information presented on BenchChem are intended solely for informational purposes. The products available for purchase on BenchChem are specifically designed for in-vitro studies, which are conducted outside of living organisms. In-vitro studies, derived from the Latin term "in glass," involve experiments performed in controlled laboratory settings using cells or tissues. It is important to note that these products are not categorized as medicines or drugs, and they have not received approval from the FDA for the prevention, treatment, or cure of any medical condition, ailment, or disease. We must emphasize that any form of bodily introduction of these products into humans or animals is strictly prohibited by law. It is essential to adhere to these guidelines to ensure compliance with legal and ethical standards in research and experimentation.