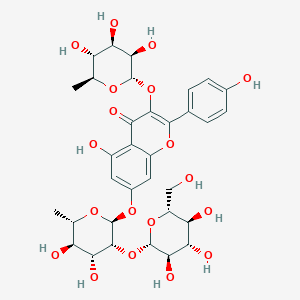
Grosvenorine
Overview
Description
Mechanism of Action
Target of Action
Grosvenorine, a main flavonoid glycoside in Siraitia grosvenorii , has been found to have numerous pharmacological effects
Mode of Action
grosvenorii, which includes this compound, can regulate physiological activities such as oxidative stress, fatty acid oxidation, and apoptosis . These interactions with its targets lead to changes in the body that contribute to its pharmacological effects .
Pharmacokinetics
ADME properties are crucial in determining the bioavailability of a compound
Result of Action
this compound has been found to have numerous pharmacological effects, including anti-diabetic, anti-tumour, anti-inflammatory, antioxidant, neuroprotective, and lipogenic inhibitory effects . These effects are the result of this compound’s interaction with its targets and the subsequent changes in the body .
Action Environment
Environmental factors can influence gene expression, which in turn can affect the action, efficacy, and stability of compounds like this compound . Factors such as light, temperature, and pollution could potentially alter the action of this compound . .
Biochemical Analysis
Biochemical Properties
Grosvenorine plays a crucial role in biochemical reactions, particularly due to its interactions with various enzymes, proteins, and other biomolecules. It has been observed to interact with enzymes involved in antioxidant activities, such as superoxide dismutase (SOD) and catalase. These interactions help in neutralizing free radicals and reducing oxidative stress within cells . Additionally, this compound has been found to interact with proteins involved in inflammatory pathways, thereby exhibiting anti-inflammatory properties.
Cellular Effects
This compound exerts significant effects on various types of cells and cellular processes. It has been shown to influence cell function by modulating cell signaling pathways, gene expression, and cellular metabolism. For instance, this compound can activate the nuclear factor erythroid 2-related factor 2 (Nrf2) pathway, leading to the upregulation of antioxidant response elements and enhancing the cell’s defense mechanisms against oxidative stress . Furthermore, this compound has been reported to inhibit the expression of pro-inflammatory cytokines, thereby reducing inflammation at the cellular level.
Molecular Mechanism
The molecular mechanism of this compound involves its binding interactions with biomolecules, enzyme inhibition or activation, and changes in gene expression. This compound binds to specific receptors on the cell surface, initiating a cascade of intracellular signaling events. It can inhibit the activity of enzymes such as cyclooxygenase (COX) and lipoxygenase (LOX), which are involved in the production of inflammatory mediators . Additionally, this compound can modulate the expression of genes related to antioxidant defense and inflammation, thereby exerting its protective effects at the molecular level.
Temporal Effects in Laboratory Settings
In laboratory settings, the effects of this compound have been observed to change over time. The compound exhibits stability under controlled conditions, but its degradation can occur under extreme pH or temperature conditions. Long-term studies have shown that this compound can maintain its antioxidant and anti-inflammatory effects over extended periods, both in vitro and in vivo . The extent of these effects may vary depending on the experimental conditions and the duration of exposure.
Dosage Effects in Animal Models
The effects of this compound vary with different dosages in animal models. At lower doses, this compound has been found to exhibit beneficial effects such as enhanced antioxidant activity and reduced inflammation. At higher doses, there may be threshold effects, leading to potential toxicity or adverse effects . It is crucial to determine the optimal dosage range to maximize the therapeutic benefits of this compound while minimizing any potential risks.
Metabolic Pathways
This compound is involved in various metabolic pathways, interacting with enzymes and cofactors that regulate metabolic flux and metabolite levels. It has been shown to enhance the activity of enzymes involved in the detoxification of reactive oxygen species (ROS), thereby reducing oxidative damage . Additionally, this compound can influence the levels of metabolites involved in inflammatory pathways, further contributing to its anti-inflammatory effects.
Transport and Distribution
Within cells and tissues, this compound is transported and distributed through specific transporters and binding proteins. These interactions facilitate its localization and accumulation in target tissues, where it can exert its biological effects . The transport and distribution of this compound are crucial for its bioavailability and therapeutic efficacy.
Subcellular Localization
This compound exhibits specific subcellular localization, which can impact its activity and function. It has been observed to localize in the cytoplasm and nucleus, where it can interact with various cellular components . Post-translational modifications and targeting signals play a role in directing this compound to specific compartments or organelles, thereby influencing its biological activity.
Preparation Methods
Synthetic Routes and Reaction Conditions: The synthesis of grosvenorine involves the extraction of the compound from the fruits of Siraitia grosvenorii. The process typically includes:
Extraction: Using solvents like ethanol or methanol to extract the flavonoids from the dried fruit.
Purification: Techniques such as column chromatography are employed to purify this compound from the crude extract.
Industrial Production Methods: Industrial production of this compound focuses on optimizing the extraction and purification processes to ensure high yield and purity. This often involves:
Large-scale extraction: Utilizing industrial solvents and advanced extraction techniques.
Purification: Employing high-performance liquid chromatography (HPLC) for large-scale purification.
Chemical Reactions Analysis
Types of Reactions: Grosvenorine undergoes various chemical reactions, including:
Oxidation: this compound can be oxidized to form different oxidation products, which may have distinct biological activities.
Reduction: Reduction reactions can modify the flavonoid structure, potentially altering its biological properties.
Substitution: Substitution reactions, particularly involving hydroxyl groups, can lead to the formation of derivatives with varied activities.
Common Reagents and Conditions:
Oxidation: Common oxidizing agents include hydrogen peroxide and potassium permanganate.
Reduction: Reducing agents like sodium borohydride are often used.
Substitution: Reagents such as acetic anhydride and sulfuric acid are employed for acetylation reactions.
Major Products: The major products formed from these reactions include various oxidized, reduced, and substituted derivatives of this compound, each with potentially unique biological activities.
Scientific Research Applications
Grosvenorine has a wide range of applications in scientific research:
Chemistry: Used as a model compound to study flavonoid chemistry and reactions.
Biology: Investigated for its role in cellular processes and its effects on various biological pathways.
Medicine: Explored for its potential therapeutic effects, including antibacterial, antioxidant, anti-inflammatory, and anti-cancer activities
Industry: Utilized in the development of natural health products and supplements due to its beneficial properties.
Comparison with Similar Compounds
Grosvenorine is unique among flavonoids due to its specific structure and biological activities. Similar compounds include:
Quercetin: Another flavonoid with strong antioxidant and anti-inflammatory properties.
Kaempferol: Known for its anticancer and cardioprotective effects.
Luteolin: Exhibits anti-inflammatory and neuroprotective activities.
Compared to these compounds, this compound stands out for its potent antibacterial activity and its specific use in traditional Chinese medicine .
Biological Activity
Grosvenorine, a bioactive compound derived from Siraitia grosvenorii, commonly known as monk fruit, has garnered significant attention due to its diverse biological activities. This article explores the pharmacological properties of this compound, including its effects on diabetes, cancer, inflammation, and its antioxidant capabilities, supported by case studies and research findings.
Chemical Composition and Sources
This compound is primarily found in Siraitia grosvenorii, which has been used traditionally in Chinese medicine and as a natural sweetener. The fruit contains several active compounds, including mogrosides, which are responsible for its sweet taste and therapeutic effects. The following table summarizes some key compounds associated with S. grosvenorii and their biological activities:
1. Anti-Diabetic Activity
This compound exhibits significant anti-diabetic properties. Research indicates that mogroside V can enhance insulin sensitivity and improve glucose metabolism. In studies involving type 2 diabetes mellitus (T2DM) models, mogroside V was shown to regulate the PI3K/Akt signaling pathway, promoting glycogen synthesis and enhancing glucose transport capacity in liver and fat tissues . Additionally, it effectively lowers blood glucose levels by inhibiting α-glucosidase activity .
2. Anti-Cancer Properties
This compound has demonstrated potential in cancer treatment. A study highlighted its ability to inhibit the migration and invasion of lung cancer cells under hyperglycemic conditions by modulating E-Cadherin and N-Cadherin expressions . This suggests that this compound may play a role in preventing metastasis in cancer patients with diabetes.
3. Anti-Inflammatory Effects
The compound exhibits notable anti-inflammatory effects. Research indicates that mogroside V can inhibit the expression of inflammatory proteins such as inducible nitric oxide synthase (iNOS) and cyclooxygenase-2 (COX-2), thereby minimizing tissue damage caused by inflammation . This property is particularly relevant in conditions characterized by chronic inflammation.
4. Antioxidant Activity
This compound is also recognized for its antioxidant properties. Studies have shown that flavonoids extracted from S. grosvenorii possess strong scavenging abilities against free radicals, significantly surpassing standard antioxidants like butylhydroxytoluene (BHT) . The antioxidant activity is attributed to the presence of phenolic hydroxyl groups that effectively neutralize reactive oxygen species (ROS) .
Case Studies
Several case studies have been conducted to evaluate the efficacy of this compound in clinical settings:
- Case Study on Diabetes Management : A clinical trial involving T2DM patients demonstrated that supplementation with mogroside V resulted in significant reductions in fasting blood glucose levels and improved insulin sensitivity over a 12-week period .
- Cancer Prevention Study : In vitro studies on lung cancer cells showed that treatment with mogroside V inhibited cell proliferation and induced apoptosis through the activation of caspase pathways .
Properties
IUPAC Name |
7-[(2S,3R,4R,5R,6S)-4,5-dihydroxy-6-methyl-3-[(2S,3R,4S,5S,6R)-3,4,5-trihydroxy-6-(hydroxymethyl)oxan-2-yl]oxyoxan-2-yl]oxy-5-hydroxy-2-(4-hydroxyphenyl)-3-[(2S,3R,4R,5R,6S)-3,4,5-trihydroxy-6-methyloxan-2-yl]oxychromen-4-one | |
---|---|---|
Source | PubChem | |
URL | https://pubchem.ncbi.nlm.nih.gov | |
Description | Data deposited in or computed by PubChem | |
InChI |
InChI=1S/C33H40O19/c1-10-19(37)23(41)26(44)31(46-10)51-29-22(40)18-15(36)7-14(8-16(18)49-28(29)12-3-5-13(35)6-4-12)48-33-30(25(43)20(38)11(2)47-33)52-32-27(45)24(42)21(39)17(9-34)50-32/h3-8,10-11,17,19-21,23-27,30-39,41-45H,9H2,1-2H3/t10-,11-,17+,19-,20-,21+,23+,24-,25+,26+,27+,30+,31-,32-,33-/m0/s1 | |
Source | PubChem | |
URL | https://pubchem.ncbi.nlm.nih.gov | |
Description | Data deposited in or computed by PubChem | |
InChI Key |
FFGGWIVHOGEVSP-NWQOLJAQSA-N | |
Source | PubChem | |
URL | https://pubchem.ncbi.nlm.nih.gov | |
Description | Data deposited in or computed by PubChem | |
Canonical SMILES |
CC1C(C(C(C(O1)OC2=C(OC3=CC(=CC(=C3C2=O)O)OC4C(C(C(C(O4)C)O)O)OC5C(C(C(C(O5)CO)O)O)O)C6=CC=C(C=C6)O)O)O)O | |
Source | PubChem | |
URL | https://pubchem.ncbi.nlm.nih.gov | |
Description | Data deposited in or computed by PubChem | |
Isomeric SMILES |
C[C@H]1[C@@H]([C@H]([C@H]([C@@H](O1)OC2=C(OC3=CC(=CC(=C3C2=O)O)O[C@H]4[C@@H]([C@@H]([C@H]([C@@H](O4)C)O)O)O[C@H]5[C@@H]([C@H]([C@@H]([C@H](O5)CO)O)O)O)C6=CC=C(C=C6)O)O)O)O | |
Source | PubChem | |
URL | https://pubchem.ncbi.nlm.nih.gov | |
Description | Data deposited in or computed by PubChem | |
Molecular Formula |
C33H40O19 | |
Source | PubChem | |
URL | https://pubchem.ncbi.nlm.nih.gov | |
Description | Data deposited in or computed by PubChem | |
Molecular Weight |
740.7 g/mol | |
Source | PubChem | |
URL | https://pubchem.ncbi.nlm.nih.gov | |
Description | Data deposited in or computed by PubChem | |
Retrosynthesis Analysis
AI-Powered Synthesis Planning: Our tool employs the Template_relevance Pistachio, Template_relevance Bkms_metabolic, Template_relevance Pistachio_ringbreaker, Template_relevance Reaxys, Template_relevance Reaxys_biocatalysis model, leveraging a vast database of chemical reactions to predict feasible synthetic routes.
One-Step Synthesis Focus: Specifically designed for one-step synthesis, it provides concise and direct routes for your target compounds, streamlining the synthesis process.
Accurate Predictions: Utilizing the extensive PISTACHIO, BKMS_METABOLIC, PISTACHIO_RINGBREAKER, REAXYS, REAXYS_BIOCATALYSIS database, our tool offers high-accuracy predictions, reflecting the latest in chemical research and data.
Strategy Settings
Precursor scoring | Relevance Heuristic |
---|---|
Min. plausibility | 0.01 |
Model | Template_relevance |
Template Set | Pistachio/Bkms_metabolic/Pistachio_ringbreaker/Reaxys/Reaxys_biocatalysis |
Top-N result to add to graph | 6 |
Feasible Synthetic Routes
Disclaimer and Information on In-Vitro Research Products
Please be aware that all articles and product information presented on BenchChem are intended solely for informational purposes. The products available for purchase on BenchChem are specifically designed for in-vitro studies, which are conducted outside of living organisms. In-vitro studies, derived from the Latin term "in glass," involve experiments performed in controlled laboratory settings using cells or tissues. It is important to note that these products are not categorized as medicines or drugs, and they have not received approval from the FDA for the prevention, treatment, or cure of any medical condition, ailment, or disease. We must emphasize that any form of bodily introduction of these products into humans or animals is strictly prohibited by law. It is essential to adhere to these guidelines to ensure compliance with legal and ethical standards in research and experimentation.