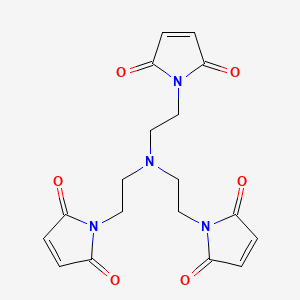
Tris(2-maleimidoethyl)amine
Overview
Description
Tris(2-maleimidoethyl)amine is an organic compound characterized by the presence of three maleimide groups attached to an amine core. This trifunctional molecule is widely used in various chemical and biological applications due to its ability to form stable covalent bonds with sulfhydryl groups. It is typically a white to pale yellow crystalline solid with high solubility in both water and organic solvents .
Preparation Methods
Synthetic Routes and Reaction Conditions: Tris(2-maleimidoethyl)amine is commonly synthesized through the reaction of maleimide with ethylenediamine. The process involves the following steps:
Reaction of Maleimide with Ethylenediamine: Maleimide is reacted with ethylenediamine under controlled conditions to form this compound. The reaction is typically carried out in an organic solvent such as dimethylformamide (DMF) or dimethyl sulfoxide (DMSO) at room temperature[][2].
Purification: The resulting product is purified through recrystallization or chromatography to obtain high-purity this compound.
Industrial Production Methods: In industrial settings, the production of this compound follows similar synthetic routes but on a larger scale. The reaction conditions are optimized to ensure high yield and purity. The use of automated reactors and purification systems enhances the efficiency and scalability of the production process[2][2].
Chemical Reactions Analysis
Types of Reactions: Tris(2-maleimidoethyl)amine primarily undergoes reactions involving its maleimide groups. These reactions include:
Addition Reactions: The maleimide groups react with sulfhydryl groups (-SH) to form stable thioether linkages.
Substitution Reactions: The maleimide groups can also participate in substitution reactions with nucleophiles, leading to the formation of various derivatives.
Common Reagents and Conditions:
Sulfhydryl Reagents: Common sulfhydryl-containing reagents include cysteine, glutathione, and thiol-containing peptides.
Reaction Conditions: The reactions are typically carried out at a pH of 6.5-7.5, with the reaction completing in 2 hours at room temperature or 4 hours at 4°C.
Major Products:
Scientific Research Applications
Tris(2-maleimidoethyl)amine has a wide range of applications in scientific research, including:
Mechanism of Action
The mechanism of action of Tris(2-maleimidoethyl)amine involves the formation of covalent bonds with sulfhydryl groups. The maleimide groups react with sulfhydryl groups to form stable thioether linkages. This reaction is highly specific and efficient, making this compound an ideal crosslinker for various applications . The molecular targets include cysteine residues in proteins and peptides, which are crucial for the formation of stable conjugates .
Comparison with Similar Compounds
Bismaleimidohexane (BMH): Bismaleimidohexane is another crosslinker containing two maleimide groups.
N,N’-Ethylenebis(maleimide): This compound contains two maleimide groups linked by an ethylene bridge.
Uniqueness of Tris(2-maleimidoethyl)amine: this compound is unique due to its trifunctional structure, which allows for the formation of three-way conjugates and complex macromolecular architectures. This property makes it highly versatile and valuable in various scientific and industrial applications .
Biological Activity
Tris(2-maleimidoethyl)amine (TMEA) is a trifunctional crosslinker known for its ability to react with thiol groups, making it a valuable tool in biochemical research and applications. Its structure, featuring three maleimide groups, allows for the conjugation of biomolecules, such as proteins and antibodies, which is crucial in various biological studies. This article will delve into the biological activity of TMEA, focusing on its interactions with multidrug resistance proteins, its applications in biosensing, and other relevant research findings.
- Chemical Formula : C₁₈H₁₈N₄O₆
- Molecular Weight : 386.36 g/mol
- CAS Number : 139112-38-2
- Solubility : Insoluble in water; soluble in DMF or DMSO.
TMEA is primarily used as a crosslinker due to its reactivity towards sulfhydryl groups, enabling the formation of stable conjugates necessary for various biochemical applications.
Interaction with Multidrug Resistance P-glycoprotein
TMEA has been shown to significantly affect the activity of human multidrug resistance P-glycoprotein (P-gp), a critical transporter involved in drug efflux and resistance mechanisms. Research indicates that:
- Cross-linking Mechanism : TMEA acts as a thiol-reactive substrate that can cross-link specific residues within the transmembrane domains of P-gp. This interaction is influenced by ATP hydrolysis, which alters the conformation of P-gp and its drug-binding pocket .
- ATPase Activity Stimulation : The presence of TMEA stimulates ATPase activity in P-gp, indicating that TMEA can enhance the transport function of this protein. Specifically, TMEA increased ATPase activity by approximately sevenfold in cysteine-less mutants of P-gp .
- Mutagenesis Studies : Cysteine-scanning mutagenesis has demonstrated that certain mutations within the transmembrane segments (TM6 and TM12) can affect how TMEA interacts with P-gp. For instance, cross-linking was observed between specific mutant pairs (e.g., L339C and V982C), suggesting that these residues are crucial for TMEA's binding and subsequent cross-linking .
Applications in Biosensing
TMEA's ability to form stable conjugates makes it suitable for use in biosensors. For instance:
- Nanowire-Based Biosensors : TMEA has been utilized in the development of immunosensors where it facilitates the covalent attachment of biomolecules to sensor surfaces. This application enhances the sensitivity and specificity of biosensors by improving signal transduction mechanisms .
Summary of Research Findings
Case Study 1: Inhibition of Drug Transport
In a study examining the interaction between TMEA and various substrates like verapamil and cyclosporin A, it was found that these drugs could inhibit TMEA-induced cross-linking. This suggests that drug binding can compete with or alter the effects of TMEA on P-gp function, highlighting its potential role in modulating drug resistance mechanisms .
Case Study 2: Enhanced Sensitivity in Immunosensors
Research involving chitosan-coated electrodes demonstrated that TMEA significantly improved the performance of immunosensors by facilitating stronger covalent bonds between antibodies and sensor surfaces. This advancement led to enhanced detection capabilities for various analytes .
Properties
IUPAC Name |
1-[2-[bis[2-(2,5-dioxopyrrol-1-yl)ethyl]amino]ethyl]pyrrole-2,5-dione | |
---|---|---|
Source | PubChem | |
URL | https://pubchem.ncbi.nlm.nih.gov | |
Description | Data deposited in or computed by PubChem | |
InChI |
InChI=1S/C18H18N4O6/c23-13-1-2-14(24)20(13)10-7-19(8-11-21-15(25)3-4-16(21)26)9-12-22-17(27)5-6-18(22)28/h1-6H,7-12H2 | |
Source | PubChem | |
URL | https://pubchem.ncbi.nlm.nih.gov | |
Description | Data deposited in or computed by PubChem | |
InChI Key |
WHEOHCIKAJUSJC-UHFFFAOYSA-N | |
Source | PubChem | |
URL | https://pubchem.ncbi.nlm.nih.gov | |
Description | Data deposited in or computed by PubChem | |
Canonical SMILES |
C1=CC(=O)N(C1=O)CCN(CCN2C(=O)C=CC2=O)CCN3C(=O)C=CC3=O | |
Source | PubChem | |
URL | https://pubchem.ncbi.nlm.nih.gov | |
Description | Data deposited in or computed by PubChem | |
Molecular Formula |
C18H18N4O6 | |
Source | PubChem | |
URL | https://pubchem.ncbi.nlm.nih.gov | |
Description | Data deposited in or computed by PubChem | |
Molecular Weight |
386.4 g/mol | |
Source | PubChem | |
URL | https://pubchem.ncbi.nlm.nih.gov | |
Description | Data deposited in or computed by PubChem | |
Retrosynthesis Analysis
AI-Powered Synthesis Planning: Our tool employs the Template_relevance Pistachio, Template_relevance Bkms_metabolic, Template_relevance Pistachio_ringbreaker, Template_relevance Reaxys, Template_relevance Reaxys_biocatalysis model, leveraging a vast database of chemical reactions to predict feasible synthetic routes.
One-Step Synthesis Focus: Specifically designed for one-step synthesis, it provides concise and direct routes for your target compounds, streamlining the synthesis process.
Accurate Predictions: Utilizing the extensive PISTACHIO, BKMS_METABOLIC, PISTACHIO_RINGBREAKER, REAXYS, REAXYS_BIOCATALYSIS database, our tool offers high-accuracy predictions, reflecting the latest in chemical research and data.
Strategy Settings
Precursor scoring | Relevance Heuristic |
---|---|
Min. plausibility | 0.01 |
Model | Template_relevance |
Template Set | Pistachio/Bkms_metabolic/Pistachio_ringbreaker/Reaxys/Reaxys_biocatalysis |
Top-N result to add to graph | 6 |
Feasible Synthetic Routes
Disclaimer and Information on In-Vitro Research Products
Please be aware that all articles and product information presented on BenchChem are intended solely for informational purposes. The products available for purchase on BenchChem are specifically designed for in-vitro studies, which are conducted outside of living organisms. In-vitro studies, derived from the Latin term "in glass," involve experiments performed in controlled laboratory settings using cells or tissues. It is important to note that these products are not categorized as medicines or drugs, and they have not received approval from the FDA for the prevention, treatment, or cure of any medical condition, ailment, or disease. We must emphasize that any form of bodily introduction of these products into humans or animals is strictly prohibited by law. It is essential to adhere to these guidelines to ensure compliance with legal and ethical standards in research and experimentation.