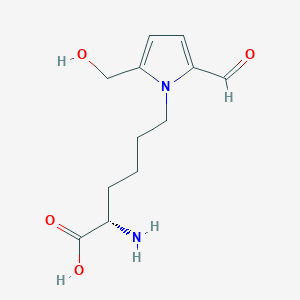
Pyrraline
Overview
Description
Pyrraline (ε-2-(formyl-5-hydroxymethyl-pyrrol-1-yl)-L-norleucine) is a major advanced glycation end product (AGE) formed during the late stages of the Maillard reaction. It arises from the reaction of 3-deoxyglucosone (3-DG), a 1,2-dicarbonyl compound derived from glucose degradation, with the ε-amino group of lysine residues in proteins or peptides . This compound is widely detected in thermally processed foods, with concentrations ranging from 12.2 mg/kg in dairy products to 242.6 mg/kg in bakery items and up to 3,680 mg/kg protein in bread crusts . Its formation is influenced by temperature, pH, and the presence of sodium ions, with optimal synthesis occurring at 140°C .
Structurally, this compound features a pyrrole ring linked to a hydroxymethyl group, making it distinct from other AGEs like carboxymethyllysine (CML) or carboxyethyllysine (CEL) . Unlike fluorescent AGEs (e.g., pentosidine), this compound is non-fluorescent and primarily exists in peptide- or protein-bound forms due to the abundance of lysine in dietary proteins .
Preparation Methods
Synthetic Routes and Reaction Conditions
Pyrraline is synthesized through the Paal–Knorr reaction, which involves the addition of 3-deoxyglucosone to the amino group of the lysine side chain, followed by the elimination of water . This reaction is typically carried out under mild conditions, often in the presence of an acid catalyst.
Industrial Production Methods
Industrial production of this compound involves the glycation of proteins, such as casein, with 3-deoxyglucosone. The modified proteins are then subjected to simulated gastrointestinal digestion to release the compound .
Chemical Reactions Analysis
Formation Pathways and Saccharide Influence
Pyrraline forms via the Maillard reaction between lysine ε-amino groups and reducing sugars. Key findings include:
-
Saccharide Reactivity Order : Formation rates vary by saccharide structure:
Lactose > Fructose > Glucose > Sucrose in lysine model systems .
Lactose-lysine systems yield the highest this compound concentrations due to enhanced reactivity under microwave heating . -
Optimal Conditions :
Saccharide | Relative this compound Yield (%) |
---|---|
Lactose | 100 (reference) |
Fructose | 85 |
Glucose | 72 |
Sucrose | 58 |
Sodium Ion (Na⁺) Modulation
Na⁺ significantly enhances this compound formation by altering reaction dynamics:
-
Concentration Dependence :
-
Mechanistic Role :
Na⁺ stabilizes Schiff base intermediates and increases 3-deoxyglucosone (3-DG) levels, a key this compound precursor .
Na⁺ (mol/L) | This compound (mmol/mol lysine) |
---|---|
0.0 | 0.06 ± 0.002 |
0.2 | 0.86 ± 0.03 |
0.5 | 0.73 ± 0.02 |
Peptide Structural Effects
Peptide sequences adjacent to lysine residues critically influence this compound formation:
-
C-Terminal Residue Impact :
-
Tripeptides vs. Dipeptides :
Tripeptides (e.g., Lys-Gly-Phe) exhibit 40–60% lower yields than analogous dipeptides .
Stability and Degradation Pathways
This compound undergoes elimination and crosslinking under prolonged heating:
-
Lysine-Mediated Instability : Excess lysine promotes this compound degradation into dithis compound and lysine-pyrraline crosslinks .
-
Temperature Sensitivity : Degradation accelerates above 160°C , reducing net this compound accumulation .
Reaction with Proteins
This compound modifies proteins, altering their functional properties:
-
Ovalbumin Glycation :
Mitigation Strategies
Encapsulating NaCl with gums or starches reduces this compound by 30–50% in model systems, highlighting methods to control AGE formation in processed foods .
Scientific Research Applications
Introduction to Pyrraline
This compound is a compound classified as an advanced glycation end product (AGE), primarily formed through the Maillard reaction between reducing sugars and amino acids, particularly lysine. Its significance has been increasingly recognized in various scientific fields, including nutrition, toxicology, and medicine. This article delves into the applications of this compound, focusing on its implications in health, food science, and potential therapeutic avenues.
Diabetes and Nephropathy
Research indicates that this compound is associated with diabetic complications, particularly nephropathy. Elevated levels of this compound-modified proteins have been detected in diabetic patients and animal models, suggesting a role in the progression of vascular damage and kidney dysfunction. A study utilizing metabolomics revealed that exposure to this compound significantly alters metabolic pathways related to amino acid metabolism, indicating its potential as a biomarker for diabetes-related complications .
Cardiovascular Diseases
This compound has been implicated in cardiovascular disorders due to its effects on protein modification and cellular interactions. The accumulation of this compound-modified albumin in macrophages has been shown to reduce the degradation of these proteins, potentially contributing to inflammation and atherosclerosis . This suggests that this compound may serve as a marker for cardiovascular health and disease progression.
Indicator of Heat Damage
In food science, this compound is used as an indicator of heat damage during food processing. Its formation is closely monitored in products subjected to high temperatures, such as baked goods and roasted meats. The presence of this compound can signify excessive heating, which may lead to undesirable health effects due to increased AGE content .
Maillard Reaction Studies
This compound's formation during the Maillard reaction has been extensively studied to understand how different conditions affect AGE production in foods. Research shows that factors such as sodium ion concentration can significantly influence the amount of this compound generated during cooking processes . This knowledge is crucial for the food industry aiming to minimize harmful AGEs while maximizing flavor and color.
Drug Development
The understanding of this compound's role in metabolic pathways opens avenues for therapeutic interventions targeting AGE-related diseases. Compounds that inhibit the formation or activity of AGEs like this compound may provide protective effects against diabetes and cardiovascular diseases .
Nutritional Interventions
Dietary strategies aimed at reducing the intake of AGEs could mitigate the health risks associated with this compound consumption. Research suggests that modifying cooking methods or ingredient choices can lower AGE levels in food products, thereby promoting better health outcomes .
Table 1: Key Findings on this compound Applications
Mechanism of Action
Pyrraline exerts its effects through the modification of proteins. It forms covalent bonds with amino acid residues, leading to changes in protein structure and function. The compound is also a substrate for the human peptide transporter 1 (PEPT1), which facilitates its absorption in the gut .
Comparison with Similar Compounds
Pyrraline belongs to a broader class of AGEs, which differ in structure, formation pathways, and biological effects. Below is a comparative analysis with key analogs:
This compound vs. Nε-(Carboxymethyl)lysine (CML) and Nε-(Carboxyethyl)lysine (CEL)
Key Differences :
- This compound is derived specifically from 3-DG, whereas CML/CEL originate from glyoxal or methylglyoxal .
- Unlike CML/CEL, this compound formation is highly sensitive to sodium ion concentrations; NaCl accelerates its synthesis by catalyzing 3-DG generation .
- This compound is less stable than CML/CEL at high temperatures, forming crosslinks with lysine residues under prolonged heating .
This compound vs. Pentosidine
Key Differences :
- Pentosidine is a cross-linking AGE with diagnostic value in aging studies, while this compound is a non-crosslinking marker of thermal processing .
- This compound is more abundant in plant-based foods (e.g., cereals), whereas pentosidine accumulates in animal-derived proteins .
This compound vs. Imidazolone
Key Differences :
- Imidazolone modifies arginine residues, unlike lysine-targeting this compound .
- This compound is more responsive to hydrophobic interactions; peptides with adjacent hydrophobic residues (e.g., Ile, Leu) enhance its formation .
Mechanistic Insights and Research Findings
- Peptide Length Impact : Dipeptides (e.g., Lys-Leu) yield 2–3× more this compound than tripeptides (e.g., Lys-Leu-Gly) due to steric hindrance in longer chains .
- Amino Acid Influence: Hydrophobic residues (e.g., Leu, Ile) adjacent to lysine increase this compound formation by 40–60% compared to polar residues (e.g., Ser, Gly) .
- Sodium Ion Catalysis : NaCl at 0.5 M boosts this compound synthesis by 70% via accelerating 3-DG formation .
- Inhibition Strategies: Phenolic extracts from highland barley reduce this compound by 49–52% via scavenging 3-DG .
Biological Activity
Pyrraline is a compound formed through the Maillard reaction, primarily derived from the interaction between glucose and lysine. It is categorized as an advanced glycation end product (AGE), which has been implicated in various biological activities and health implications, particularly in relation to chronic diseases such as diabetes and cardiovascular conditions. This article delves into the biological activity of this compound, supported by data tables, case studies, and research findings.
This compound is formed when reducing sugars react with amino acids, particularly lysine, during cooking or processing of food. This reaction not only enhances flavor but also generates various AGEs that can have detrimental effects on health. This compound's structure allows it to participate in further reactions, potentially leading to more complex AGEs.
Biological Implications
- Diabetes and Cardiovascular Disease : this compound has been identified as a causative factor in the pathogenesis of diabetes and cardiovascular diseases. Elevated levels of this compound are associated with increased risk factors for these conditions due to its role in promoting oxidative stress and inflammation .
- Advanced Glycation End Products (AGEs) : As an AGE, this compound contributes to the formation of cross-links in proteins, which can impair their function. This is particularly evident in the extracellular matrix of tissues affected by diabetes and aging .
- Cellular Effects : Research has shown that this compound can activate specific transcription factors such as Nrf2, which plays a crucial role in cellular defense against oxidative stress. Studies on human colon cancer cells demonstrated that protein-bound this compound can influence cellular responses differently than its free form .
Study on Peptide-Bound this compound
A study analyzed the formation of peptide-bound this compound from various protein sources. The results indicated significant variability in the levels of this compound depending on the source and processing conditions:
Sample | Peptide Source | Free-Form this compound (mg/100 g Protein) | Peptide Bound this compound (mg/100 g Protein) | Total this compound (mg/100 g Protein) |
---|---|---|---|---|
PED1 | WPH | nd | 45.23 ± 1.95 | 45.23 ± 1.95 |
PED2 | WPH | 5.60 ± 1.15 | 76.05 ± 0.60 | 81.70 ± 0.38 |
PED3 | WPH | tr | 52.65 ± 2.30 | 52.65 ± 2.30 |
PED4 | WPH | nd | 59.15 ± 1.58 | 59.15 ± 1.58 |
PED5 | WPH | tr | 56.10 ± 0.30 | 56.10 ± 0.30 |
*nd = not detected; tr = trace .
Immunohistochemical Studies
Immunohistochemical localization studies revealed that this compound is predominantly found in the sclerosed extracellular matrix of renal tissues from diabetic individuals, indicating its potential role as a biomarker for kidney damage related to diabetes .
Kinetic Studies on Formation
Research on the kinetics of peptide-bound this compound formation highlighted that varying reactant concentrations significantly influence the amount of this compound produced during the Maillard reaction . This finding underscores the importance of processing conditions in determining the biological activity of this compound.
Q & A
Basic Research Questions
Q. What experimental conditions optimize pyrraline formation in model systems, and how are these parameters validated?
this compound formation can be optimized using response surface methodology (RSM) combined with one-factor-at-a-time experiments. For example, in a lysine-glucose (Lys-Glu) model system, key parameters include heating time (50 min), temperature (110°C), pH (7.4), and molar ratios (Lys: 0.6 mol/L, Glu: 0.5 mol/L), yielding a maximum this compound concentration of 4.69 μmol/L. Validation involves high-performance liquid chromatography (HPLC) for quantification and Box-Behnken experimental designs to assess interactions between variables .
Q. What analytical methods are most reliable for detecting and quantifying this compound in food and biological samples?
HPLC with UV or fluorescence detection is the gold standard for this compound quantification due to its sensitivity and specificity. For complex matrices (e.g., milk powders), molecularly imprinted polymers (MIPs) coupled with luminescent metal-organic frameworks (MOFs) enhance selectivity. In biological tissues (e.g., cardiac membranes), autoradiography and Western blotting are used to detect this compound adducts, with statistical validation via methods like ANOVA to ensure reproducibility .
Q. Which factors most significantly influence this compound formation during food processing?
Critical factors include:
- Temperature and time : Prolonged heating (>100°C) accelerates Maillard reactions.
- pH : Neutral to slightly alkaline conditions (pH 7–8) favor this compound synthesis.
- Reactant ratios : Excess lysine relative to reducing sugars (e.g., glucose) increases yield. Experimental designs should incorporate factorial analyses to isolate variable effects .
Q. How can researchers formulate focused research questions for this compound studies?
Use the PICOT framework:
- Population/Problem : E.g., "In glycated proteins (P)...
- Intervention/Indicator : ...how does thermal processing (I)...
- Comparison : ...compared to non-thermal methods (C)...
- Outcome : ...affect this compound adduct formation (O)?" This ensures alignment with hypotheses and methodological feasibility .
Advanced Research Questions
Q. How do this compound-protein adducts alter molecular interactions in vivo, and what methods elucidate these mechanisms?
this compound adducts on proteins (e.g., cardiac ryanodine receptors) can disrupt calcium signaling. Techniques like co-immunoprecipitation and molecular docking simulations are used to study binding affinities. In diabetic rat models, comparative autoradiography (control vs. treated groups) reveals adduct accumulation, validated via p-values (<0.05) to confirm statistical significance .
Q. What contradictions exist in this compound’s role as a biomarker for dietary glycation, and how can they be resolved?
Discrepancies arise in correlating dietary this compound intake with tissue adduct levels. For instance, some studies report linear dose-response relationships, while others note saturation effects. Meta-analyses using random-effects models and subgroup analyses (e.g., diabetic vs. non-diabetic cohorts) can reconcile these differences. Additionally, isotopic labeling (e.g., ¹³C-glucose) helps trace this compound origins in vivo .
Q. What novel methodologies improve the sensitivity of this compound detection in heterogeneous samples?
Dual-ligand MOF-based sensors enhance specificity by leveraging π-π stacking and hydrogen bonding with this compound. For example, Eu³⁺-MOFs functionalized with MIPs achieve detection limits of 0.1 nM in milk. Cross-validation with LC-MS/MS is critical to avoid false positives from structural analogs .
Q. How can in vitro findings on this compound toxicity be extrapolated to human health contexts?
Use physiologically based kinetic (PBK) modeling to simulate human exposure. For instance, IC₅₀ values from cell cultures (e.g., endothelial cells) are adjusted for bioavailability and metabolic clearance rates. Cohort studies with urinary this compound excretion as a proxy further validate these models .
Q. What statistical approaches are optimal for analyzing this compound formation data with high variability?
Multivariate ANOVA (MANOVA) accounts for interdependent variables (e.g., pH, temperature). For non-linear relationships, generalized additive models (GAMs) or machine learning algorithms (e.g., random forests) identify hidden patterns. Sensitivity analyses quantify parameter uncertainty .
Q. How do this compound adducts interact with cellular repair mechanisms, and what experimental designs capture these dynamics?
Time-course experiments with pulse-chase labeling track adduct turnover in cell lines. Knockout models (e.g., siRNA targeting proteasomal subunits) assess degradation pathways. Confocal microscopy with fluorescent antibodies visualizes adduct localization, while RNA-seq identifies downstream gene expression changes .
Q. Methodological Guidelines
- Data Contradiction Analysis : Compare results with prior studies using funnel plots to detect publication bias. For conflicting hypotheses (e.g., this compound as a biomarker vs. bystander metabolite), apply Bradford Hill criteria for causality .
- Experimental Replicability : Report detailed protocols via platforms like Protocols.io . Include negative controls (e.g., aminoguanidine-treated samples) to confirm this compound-specific effects .
- Ethical Compliance : For human studies, obtain IRB approval and document informed consent. Use de-identified data repositories for transparency .
Properties
IUPAC Name |
2-amino-6-[2-formyl-5-(hydroxymethyl)pyrrol-1-yl]hexanoic acid | |
---|---|---|
Details | Computed by LexiChem 2.6.6 (PubChem release 2019.06.18) | |
Source | PubChem | |
URL | https://pubchem.ncbi.nlm.nih.gov | |
Description | Data deposited in or computed by PubChem | |
InChI |
InChI=1S/C12H18N2O4/c13-11(12(17)18)3-1-2-6-14-9(7-15)4-5-10(14)8-16/h4-5,7,11,16H,1-3,6,8,13H2,(H,17,18) | |
Details | Computed by InChI 1.0.5 (PubChem release 2019.06.18) | |
Source | PubChem | |
URL | https://pubchem.ncbi.nlm.nih.gov | |
Description | Data deposited in or computed by PubChem | |
InChI Key |
VTYFITADLSVOAS-UHFFFAOYSA-N | |
Details | Computed by InChI 1.0.5 (PubChem release 2019.06.18) | |
Source | PubChem | |
URL | https://pubchem.ncbi.nlm.nih.gov | |
Description | Data deposited in or computed by PubChem | |
Canonical SMILES |
C1=C(N(C(=C1)C=O)CCCCC(C(=O)O)N)CO | |
Details | Computed by OEChem 2.1.5 (PubChem release 2019.06.18) | |
Source | PubChem | |
URL | https://pubchem.ncbi.nlm.nih.gov | |
Description | Data deposited in or computed by PubChem | |
Molecular Formula |
C12H18N2O4 | |
Details | Computed by PubChem 2.1 (PubChem release 2019.06.18) | |
Source | PubChem | |
URL | https://pubchem.ncbi.nlm.nih.gov | |
Description | Data deposited in or computed by PubChem | |
Molecular Weight |
254.28 g/mol | |
Details | Computed by PubChem 2.1 (PubChem release 2021.05.07) | |
Source | PubChem | |
URL | https://pubchem.ncbi.nlm.nih.gov | |
Description | Data deposited in or computed by PubChem | |
CAS No. |
74509-14-1 | |
Record name | Pyrraline | |
Source | Human Metabolome Database (HMDB) | |
URL | http://www.hmdb.ca/metabolites/HMDB0033143 | |
Description | The Human Metabolome Database (HMDB) is a freely available electronic database containing detailed information about small molecule metabolites found in the human body. | |
Explanation | HMDB is offered to the public as a freely available resource. Use and re-distribution of the data, in whole or in part, for commercial purposes requires explicit permission of the authors and explicit acknowledgment of the source material (HMDB) and the original publication (see the HMDB citing page). We ask that users who download significant portions of the database cite the HMDB paper in any resulting publications. | |
Retrosynthesis Analysis
AI-Powered Synthesis Planning: Our tool employs the Template_relevance Pistachio, Template_relevance Bkms_metabolic, Template_relevance Pistachio_ringbreaker, Template_relevance Reaxys, Template_relevance Reaxys_biocatalysis model, leveraging a vast database of chemical reactions to predict feasible synthetic routes.
One-Step Synthesis Focus: Specifically designed for one-step synthesis, it provides concise and direct routes for your target compounds, streamlining the synthesis process.
Accurate Predictions: Utilizing the extensive PISTACHIO, BKMS_METABOLIC, PISTACHIO_RINGBREAKER, REAXYS, REAXYS_BIOCATALYSIS database, our tool offers high-accuracy predictions, reflecting the latest in chemical research and data.
Strategy Settings
Precursor scoring | Relevance Heuristic |
---|---|
Min. plausibility | 0.01 |
Model | Template_relevance |
Template Set | Pistachio/Bkms_metabolic/Pistachio_ringbreaker/Reaxys/Reaxys_biocatalysis |
Top-N result to add to graph | 6 |
Feasible Synthetic Routes
Disclaimer and Information on In-Vitro Research Products
Please be aware that all articles and product information presented on BenchChem are intended solely for informational purposes. The products available for purchase on BenchChem are specifically designed for in-vitro studies, which are conducted outside of living organisms. In-vitro studies, derived from the Latin term "in glass," involve experiments performed in controlled laboratory settings using cells or tissues. It is important to note that these products are not categorized as medicines or drugs, and they have not received approval from the FDA for the prevention, treatment, or cure of any medical condition, ailment, or disease. We must emphasize that any form of bodily introduction of these products into humans or animals is strictly prohibited by law. It is essential to adhere to these guidelines to ensure compliance with legal and ethical standards in research and experimentation.