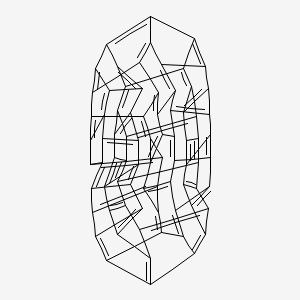
Fullerene C78
Overview
Description
Fullerene C78, a member of the higher fullerenes (Cₙ, n > 70), was first isolated in 1991 alongside C76 and C84 via high-performance liquid chromatography (HPLC) . Structurally, C78 adheres to the isolated pentagon rule (IPR), which minimizes adjacent pentagons to enhance stability. Five IPR-compliant isomers are theoretically possible for C78, but only four have been experimentally characterized:
- C78(1) (D₃ symmetry): The first isolated isomer, identified via ¹³C NMR .
- C78(2) and C78(3) (C₂ᵥ symmetry): Co-produced in arc-discharge soot and separated chromatographically .
- C78(5) (D₃ₕ symmetry): Synthesized as a trifluoromethyl derivative (C78(CF₃)₁₂), confirming its structure .
C78 exhibits a HOMO-LUMO gap of 2.47 eV (for C78(4)), comparable to C70 (2.69 eV) and C60 (2.76 eV), suggesting moderate electronic stability . Its synthesis typically occurs via thermal plasma methods with metallic/non-metallic additives (e.g., Ti, B) that enhance yields of higher fullerenes .
Preparation Methods
Arc Discharge Synthesis of Fullerene C78
Principles of Arc Discharge Technology
The arc discharge method, first employed for fullerene production in 1990, remains a cornerstone for synthesizing higher fullerenes like C78. This technique involves vaporizing graphite electrodes in a low-pressure inert gas atmosphere (typically helium or argon) to generate carbon plasma, which condenses into soot containing fullerenes . The yield of C78 in this process is influenced by parameters such as discharge current, chamber pressure, and electrode configuration.
Recent advancements have integrated resistive heating with traditional arc discharge to enhance yields. By resistively heating one electrode to 2,500–3,000°C before initiating the arc, researchers achieved a 67% increase in fullerene production compared to conventional setups . This hybrid approach promotes more efficient carbon vaporization and reduces energy consumption.
Optimization of Process Parameters
Key parameters for optimizing C78 synthesis include:
-
Discharge Current : Higher currents (150–200 A) increase vaporization rates but may favor smaller fullerenes like C60.
-
Chamber Pressure : Optimal pressures range from 100–200 Torr, balancing carbon condensation and fullerene stability .
-
Electrode Geometry : Larger chamber diameters (e.g., 140 mm) improve yield by extending plasma residence time .
A study using a chamber with a 140 mm diameter and 500 mm length reported C78 yields of 6–8% in the extracted soot, compared to 3–4% in smaller systems . Post-synthesis purification via high-performance liquid chromatography (HPLC) is critical for isolating C78 isomers from mixtures dominated by C60 and C70 .
Table 1: Arc Discharge Conditions and C78 Yields
Discharge Current (A) | Chamber Pressure (Torr) | Chamber Diameter (mm) | C78 Yield (%) |
---|---|---|---|
150 | 100 | 140 | 8.0 |
150 | 200 | 140 | 6.0 |
200 | 100 | 100 | 4.5 |
Precursor-Based Synthesis of this compound
Design of Fullerene Precursors
Precursor-based synthesis offers a selective route to C78 by using polycyclic aromatic hydrocarbons (PAHs) that template the fullerene cage. A seminal study demonstrated the synthesis of a C78 precursor derived from truxenone, a PAH with a coronene-like structure . This precursor contains 78 carbon atoms pre-arranged in positions corresponding to the target fullerene, with 93 of the 117 required C–C bonds already formed.
Flash Vacuum Pyrolysis (FVP)
The precursor undergoes flash vacuum pyrolysis at 1,000–1,100°C, inducing tandem cyclization to form the C78 cage. This method minimizes Stone–Wales rearrangements and C2 loss, preserving the desired isomer . The absence of competing isomers in the product simplifies purification, addressing a major limitation of arc discharge.
Table 2: FVP Conditions for C78 Synthesis
Precursor Structure | Temperature (°C) | Pressure (mbar) | Selectivity for C78 (%) |
---|---|---|---|
Truxenone derivative | 1,100 | 10⁻³ | >90 |
Challenges and Advancements
While precursor-based synthesis achieves high selectivity, the multi-step preparation of PAH precursors remains labor-intensive. Recent work has explored fluorinated precursors to stabilize reactive intermediates, though scalability remains a hurdle .
Liquid-Liquid Interfacial Precipitation (LLIP)
Formation of C78 Microstructures
The LLIP method enables the growth of single-crystal C78 microstructures by exploiting solubility differences at the interface of immiscible solvents. A 2021 study reported the synthesis of cubic and dice-like C78 crystals using trimethylbenzene (TMB) and isopropanol . At a TMB-to-isopropanol ratio of 1:3, microcubes with edge lengths of 5–10 µm formed within 48 hours. Agitating the solution transformed these cubes into porous dice-like structures, enhancing surface area for optoelectronic applications .
Structural and Functional Characterization
X-ray diffraction confirmed a simple cubic lattice (a = 10.6 Å) with TMB molecules intercalated between C78 cages. Photoluminescence studies revealed a 40% increase in quantum yield compared to amorphous C78 powder, attributed to reduced defect density in the microcrystals .
Table 3: LLIP Parameters and Crystal Properties
Solvent Ratio (TMB:IPA) | Crystallization Time (h) | Microstructure Type | PL Intensity (a.u.) |
---|---|---|---|
1:3 | 48 | Cubic | 320 |
1:3 (agitated) | 48 | Dice-like | 450 |
Comparative Analysis of Synthesis Methods
Yield and Selectivity
-
Arc Discharge : High throughput but low selectivity (C78 ≤8% of soot) .
-
Precursor-Based : Near-complete selectivity but low scalability .
-
LLIP : Produces functional materials but requires pre-synthesized C78 .
Industrial Applicability
Arc discharge is the most scalable method, with yields sufficient for commercial production. However, the energy-intensive nature of resistive heating and the need for HPLC purification limit cost-effectiveness. Precursor-based synthesis, while selective, is currently confined to laboratory-scale applications due to complex precursor synthesis.
Chemical Reactions Analysis
Fullerene C78 undergoes various chemical reactions, including:
Substitution: This compound can undergo nucleophilic substitution reactions, where nucleophiles replace hydrogen atoms on the fullerene cage.
Cycloaddition: This involves the addition of cyclic compounds to the fullerene structure, forming new ring systems.
Common reagents used in these reactions include chlorobenzene, aluminium chloride, and various nucleophiles . The major products formed from these reactions are fullerene derivatives with enhanced solubility and reactivity .
Scientific Research Applications
Structural and Chemical Properties
Fullerene C78 exhibits a distinct molecular structure characterized by its spherical shape, which allows for various isomer formations. Notably, two isomers of C78 have been isolated, showcasing different structural configurations that influence their chemical behavior and potential applications . The unique electron-accepting properties of fullerenes make them suitable for various electronic applications .
Applications in Nanotechnology
This compound finds extensive use in nanotechnology due to its nanoscale dimensions and unique properties. Key applications include:
- Nanomaterials : this compound can be utilized in the development of advanced nanomaterials, enhancing the mechanical and thermal properties of composites .
- Hydrogen Storage : Research indicates that C78 can encapsulate hydrogen molecules, making it a promising candidate for hydrogen storage solutions, which are crucial for energy applications .
Pharmaceutical Applications
The antioxidant properties of this compound have led to its exploration in the pharmaceutical field. Studies suggest that fullerenes can act as effective antioxidants, potentially aiding in the treatment of diseases such as cancer and neurodegenerative disorders .
Case Study: Antiviral Properties
A study highlighted the antiviral capabilities of fullerenes, suggesting that their unique structure allows them to interact with viral particles effectively. This interaction can inhibit viral replication, showcasing the potential for fullerene-based antiviral drugs .
Electronics and Photovoltaics
This compound's ability to accept electrons makes it an attractive material for use in electronic devices:
- Organic Photovoltaics : Fullerene derivatives, including C78, have been investigated as electron-transporting materials in organic solar cells. Their incorporation into photovoltaic systems has shown improved efficiency compared to traditional materials .
- Thin-Film Transistors : The electron-accepting characteristics of C78 enhance its performance in organic thin-film transistors, contributing to advancements in flexible electronics .
Catalysis
Research has indicated that this compound can serve as a catalyst or catalyst support in various chemical reactions. Its unique structure allows for increased surface area and reactivity, making it suitable for catalytic applications in organic synthesis and environmental remediation .
Mechanism of Action
The mechanism by which Fullerene C78 exerts its effects involves its ability to interact with various molecular targets and pathways. Its unique structure allows it to act as an electron acceptor, facilitating electron transfer reactions . This property is particularly useful in applications like organic solar cells, where this compound can enhance the efficiency of light absorption and energy conversion .
Comparison with Similar Compounds
Key Findings :
- C78 is less abundant than C60/C70 but more accessible than C84 due to fewer isomers .
- Additives like boron increase C78 yield by 30% compared to C76 .
Structural and Stability Comparisons
Key Findings :
- C78 isomers (e.g., C78:2/C78:3) have lower Clar numbers than C60/C70, correlating with reduced aromatic stability .
- The elusive C78(4) (D₃ₕ) is 0.2 eV less stable than C78(1) but energetically comparable to C70 .
Electronic and Spectral Properties
Property | C78 | C70 | C76 | C84 |
---|---|---|---|---|
HOMO-LUMO Gap (eV) | 2.47 (C78(4)) | 2.69 | 1.98 | 1.85 |
Ionization Potential | 7.1 eV | 7.6 eV | 7.0 eV | 6.8 eV |
UV-Vis Peaks | 330 nm (broad) | 340 nm (sharp) | 325 nm (broad) | 310 nm (broad) |
Key Findings :
- C78's radical cation spectrum in oleum shows distinct NIR absorption at 1050 nm, differing from C70 (980 nm) and C84 (1100 nm) .
- The HOMO-LUMO gap of C78(4) (2.47 eV) bridges the electronic properties of C60 and smaller fullerenes like C84 .
Key Findings :
Biological Activity
Fullerene C78, a member of the fullerene family, exhibits a range of biological activities that have garnered significant interest in various fields, including medicine and materials science. This article provides a comprehensive overview of the biological properties of C78, supported by research findings, case studies, and data tables.
Structure and Properties
This compound is characterized by its unique molecular structure, consisting of 78 carbon atoms arranged in a cage-like formation. The compound can exist in numerous isomeric forms, with theoretical predictions suggesting over 20,000 possible structures. However, only a limited number of these isomers have been synthesized and studied .
Biological Activities
1. Antioxidant Properties
Fullerenes, including C78, are known for their ability to act as antioxidants. They can scavenge free radicals and reactive oxygen species (ROS), which are implicated in various degenerative diseases. Studies have shown that water-soluble derivatives of fullerenes demonstrate significant antioxidant activity comparable to natural antioxidants like Vitamin E .
2. Antimicrobial Activity
Research indicates that this compound exhibits antimicrobial properties. The mechanism is believed to involve disruption of bacterial cell membranes through insertion into lipid bilayers, leading to increased permeability and cell death . This property has potential applications in developing antimicrobial agents.
3. Antiviral Activity
Fullerene derivatives have been investigated for their antiviral effects, particularly against HIV. Molecular modeling studies suggest that fullerene structures can interact with viral proteases, inhibiting their activity. Experimental assays have confirmed the antiviral efficacy of fullerene derivatives against HIV-1 and HIV-2 .
4. Photodynamic Therapy
C78 has potential applications in photodynamic therapy (PDT) due to its ability to generate singlet oxygen upon photoexcitation. This property can be harnessed for targeted cancer therapies by conjugating fullerenes with specific agents that interact with nucleic acids or proteins .
Case Studies
Case Study 1: Neuroprotective Effects
A study demonstrated that a water-soluble derivative of this compound could protect neuronal cells from oxidative stress-induced apoptosis. The compound was shown to prevent cell death in cortical cultures exposed to neurotoxic agents without interfering with calcium influx pathways .
Case Study 2: Antiviral Efficacy
In vitro experiments revealed that fullerene derivatives could inhibit HIV replication in infected cell lines. The study highlighted the potential of C78 as a scaffold for developing new antiviral drugs targeting HIV protease .
Data Tables
Biological Activity | Mechanism | Reference |
---|---|---|
Antioxidant | Scavenging ROS | |
Antimicrobial | Membrane disruption | |
Antiviral | Protease inhibition | |
Photodynamic therapy | Singlet oxygen generation |
Research Findings
Recent studies have focused on the synthesis and characterization of this compound and its derivatives. Notably:
- Synthesis Techniques: Various methods such as flash pyrolysis and chemical vapor deposition have been employed to synthesize C78, allowing for the exploration of its biological properties .
- Toxicity Assessments: Initial concerns regarding the toxicity of fullerene aggregates were attributed to by-products from synthesis methods rather than the fullerenes themselves. Subsequent investigations confirmed that properly synthesized fullerenes exhibit low toxicity in biological systems .
Q & A
Basic Research Questions
Q. What experimental methods are used to isolate and characterize different isomers of C78?
Isolation of C78 isomers typically employs high-performance liquid chromatography (HPLC) due to their distinct solubility and retention properties. For example, C78(1) (D3 symmetry) and C78(2/3) (C2v symmetry) are separated via toluene/hexane solvent systems and confirmed using ¹³C NMR spectroscopy . Structural characterization relies on single-crystal X-ray crystallography, as demonstrated for trifluoromethylated derivatives like C78(2)(CF₃)₁₀ and C78(3)(CF₃)₁₂, which reveal addition patterns and cage geometries .
Q. How does the isolated pentagon rule (IPR) govern the stability of C78 isomers?
The IPR dictates that stable fullerene isomers minimize adjacent pentagons. For C78, five IPR-compliant isomers exist: D3-C78(1), C2v-C78(2/3), and D3h-C78(4/5). C78(4) (D3h) is the least stable due to higher strain, while C78(1–3) are more abundant in soot extracts. Stability correlates with HOMO-LUMO gaps; for instance, C78(1) has a gap of ~1.5 eV, comparable to C70 .
Q. What synthesis conditions favor selective formation of specific C78 isomers?
Pressure-controlled graphite vaporization influences isomer distribution. For example, increasing inert gas pressure during synthesis enhances the yield of C2v’-C78(2) from 0% to 50%. This selectivity arises from kinetic control during cage formation rather than post-synthesis isomerization .
Advanced Research Questions
Q. How do discrepancies between theoretical predictions and experimental data for C78 electronic properties arise?
Density functional theory (DFT) often overestimates HOMO-LUMO gaps. For C78(4), DFT predicts a gap of 2.47 eV, but experimental data (e.g., electrochemical studies) suggest smaller gaps due to solvent effects and intermolecular interactions. Such gaps are measured via Osteryoung square wave voltammetry (OSWV), revealing oxidation/reduction potentials that vary between isomers .
Q. What methodological challenges exist in resolving structural ambiguities of low-solubility C78 isomers like D3h-C78(5)?
D3h-C78(5) is poorly soluble, necessitating derivatization (e.g., trifluoromethylation) for isolation. Structural confirmation requires synchrotron X-ray crystallography, as seen in C78(5)(CF₃)₁₂, where CF₃ groups stabilize the cage and enable diffraction analysis . Trapped ion mobility spectrometry (TIMS) offers an alternative, distinguishing isomers via collisional cross-section (CCS) differences (e.g., ΔCCS = 1 Ų between C78(1) and C78(2)) .
Q. How do Stone-Wales rearrangements (SWRs) impact the isomerization pathways of C78?
SWRs mediate interconversion between C78(2–5) isomers but not C78(1). For example, C78(2) (C2v) can transform into D3h-C78(5) via pyracylene-type bond rotations. However, SWRs are kinetically hindered at room temperature, as shown by pressure-dependent synthesis studies .
Q. What computational strategies reconcile contradictions in NMR data for C78 derivatives?
Theoretical ¹³C NMR chemical shift calculations (using spiral algorithms and DFT) match experimental data to assign cage symmetries. For example, C78(1)(CF₃)₁₄ exhibits distinct NMR signals due to its D3 symmetry, contrasting with C2v isomers . Discrepancies in peak intensities often arise from dynamic Jahn-Teller distortions in solution .
Q. How do endohedral clusters (e.g., Sc₃N@C78) alter host-guest interaction dynamics compared to empty C78?
Encapsulation of Sc₃N increases the electron affinity of C78, shifting its redox potentials. Computational studies show that Sc₃N@C78 exhibits stronger π-π interactions with concave hosts (e.g., corannulene) due to charge transfer, unlike empty C78, which relies on van der Waals forces .
Q. Methodological Notes
- Data Contradictions : Electrochemical data for C78 isomers may overlap (e.g., oxidation peaks at 0.70–0.95 V), requiring tandem techniques like HPLC-OSWV for deconvolution .
- Advanced Separation : TIMS outperforms HPLC in speed and theoretical comparability but requires calibration with DFT-derived CCS values .
Properties
IUPAC Name |
tetracontacyclo[25.6.5.516,28.44,32.35,11.321,34.28,10.212,15.222,35.229,31.113,20.124,38.02,6.014,19.017,25.018,23.030,37.033,36.547,54.446,53.448,58.126,51.150,52.03,45.07,42.09,61.039,40.041,43.044,63.049,76.055,78.056,62.057,68.059,64.060,67.065,69.066,71.070,73.072,75.074,77]octaheptaconta-1,3(45),4(48),5(61),6,8,10,12,14,16,18,20,22,24(39),25,27(38),28,30,32,34(42),35(40),36,41(43),44(63),46,49(76),50(77),51,53,55(78),56(62),57,59,64,66,68,70(73),71,74-nonatriacontaene | |
---|---|---|
Source | PubChem | |
URL | https://pubchem.ncbi.nlm.nih.gov | |
Description | Data deposited in or computed by PubChem | |
InChI |
InChI=1S/C78/c1-2-6-14-30-22-10(2)26-25-9(1)21-29-13-5(1)17-18(6)38-34(14)58-72-50(30)66-42(22)54-46(26)69-45(25)53-41(21)65-49(29)71-57-33(13)37(17)61-62(38)78-64-40-20-8-4-3-7-15-31-23-11(3)27-28-12(4)24-32-16(8)36(40)60(76(58)78)74(72)52(32)68(66)44(24)56(54)48(28)70(69)47(27)55(53)43(23)67(65)51(31)73(71)59-35(15)39(19(7)20)63(64)77(61)75(57)59 | |
Source | PubChem | |
URL | https://pubchem.ncbi.nlm.nih.gov | |
Description | Data deposited in or computed by PubChem | |
InChI Key |
VELSFHQDWXAPNK-UHFFFAOYSA-N | |
Source | PubChem | |
URL | https://pubchem.ncbi.nlm.nih.gov | |
Description | Data deposited in or computed by PubChem | |
Canonical SMILES |
C12=C3C4=C5C6=C7C8=C9C%10=C%11C%12=C%13C%14=C%15C%16=C%12C%12=C%17C%18=C%16C%16=C%19C%20=C(C1=C5C(=C%18%20)C%17=C6C8=C%11%12)C1=C5C%19=C6C8=C%11C5=C5C(=C21)C1=C3C2=C3C%12=C1C1=C5C%11=C5C%11=C8C(=C%14C8=C%11C%11=C%14C(=C%12C1=C%115)C1=C3C(=C9C3=C1C%14=C8C%13=C%103)C7=C42)C6=C%16%15 | |
Source | PubChem | |
URL | https://pubchem.ncbi.nlm.nih.gov | |
Description | Data deposited in or computed by PubChem | |
Molecular Formula |
C78 | |
Source | PubChem | |
URL | https://pubchem.ncbi.nlm.nih.gov | |
Description | Data deposited in or computed by PubChem | |
Molecular Weight |
936.8 g/mol | |
Source | PubChem | |
URL | https://pubchem.ncbi.nlm.nih.gov | |
Description | Data deposited in or computed by PubChem | |
Disclaimer and Information on In-Vitro Research Products
Please be aware that all articles and product information presented on BenchChem are intended solely for informational purposes. The products available for purchase on BenchChem are specifically designed for in-vitro studies, which are conducted outside of living organisms. In-vitro studies, derived from the Latin term "in glass," involve experiments performed in controlled laboratory settings using cells or tissues. It is important to note that these products are not categorized as medicines or drugs, and they have not received approval from the FDA for the prevention, treatment, or cure of any medical condition, ailment, or disease. We must emphasize that any form of bodily introduction of these products into humans or animals is strictly prohibited by law. It is essential to adhere to these guidelines to ensure compliance with legal and ethical standards in research and experimentation.