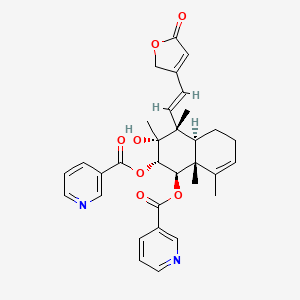
Scutebarbatine A
Overview
Description
Mechanism of Action
Target of Action
Scutebarbatine A, a major diterpenoid produced in the leaves of Scutellaria barbata , primarily targets cancer cells . It specifically induces apoptosis in human colon cancer cells and lung carcinoma A549 cells The major class of proteins down-regulated by this compound are pro-survival proteins, the Inhibitors of Apoptosis (IAPs), and IAP regulating proteins .
Mode of Action
This compound interacts with its targets by inhibiting the IAPs . This inhibition releases the molecular brakes (the IAPs) on apoptosis in cell death-evading cancer cells . It also triggers apoptosis via the activation of MAPK and ER stress .
Biochemical Pathways
This compound affects the apoptosis pathway in cancer cells. It up-regulates the expressions of cytochrome c, caspase-3 and 9, and down-regulates the levels of Bcl-2 . These changes lead to the induction of apoptosis, specifically in cancer cells .
Result of Action
The result of this compound’s action is the induction of apoptosis, specifically in cancer cells . This is achieved through the up-regulation of cytochrome c, caspase-3 and 9, and the down-regulation of Bcl-2 . It has a notable antitumor effect on A549 cancer via mitochondria-mediated apoptosis .
Biochemical Analysis
Biochemical Properties
Scutebarbatine A plays a crucial role in biochemical reactions by interacting with several key biomolecules. It induces dose-dependent apoptosis specifically in cancer cells by down-regulating pro-survival proteins, such as Inhibitors of Apoptosis (IAPs), and IAP-regulating proteins . Additionally, this compound interacts with enzymes like caspase-3 and caspase-9, up-regulating their expressions and promoting apoptosis . The compound also affects the expression of cytochrome c and Bcl-2, further facilitating the apoptotic process .
Cellular Effects
This compound exerts significant effects on various types of cells and cellular processes. In human lung carcinoma A549 cell lines, it induces apoptosis in a concentration-dependent manner . The compound influences cell function by activating the p38 mitogen-activated protein kinase (MAPK) pathway and inducing endoplasmic reticulum (ER) stress . These actions lead to changes in cell signaling pathways, gene expression, and cellular metabolism, ultimately resulting in cell death.
Molecular Mechanism
At the molecular level, this compound exerts its effects through several mechanisms. It binds to and inhibits pro-survival proteins, releasing the molecular brakes on apoptosis in cancer cells . The compound also activates the caspase cascade, leading to the cleavage of key cellular proteins and the execution of apoptosis . Furthermore, this compound induces ER stress and activates the MAPK pathway, contributing to its cytotoxic effects .
Temporal Effects in Laboratory Settings
The effects of this compound change over time in laboratory settings. Studies have shown that the compound induces substantial apoptosis in human colon cancer Caco-2 cells within 24 hours of incubation . The stability and degradation of this compound in laboratory settings are crucial factors that influence its long-term effects on cellular function. In vitro and in vivo studies have demonstrated that this compound maintains its antitumor activity over extended periods, although its efficacy may decrease due to degradation .
Dosage Effects in Animal Models
The effects of this compound vary with different dosages in animal models. In studies involving transplanted tumor nude mice, this compound exhibited significant antitumor effects at various dosages . Higher doses of the compound may lead to toxic or adverse effects, highlighting the importance of determining the optimal therapeutic dosage. Threshold effects have been observed, where lower doses may not induce significant apoptosis, while higher doses effectively trigger cell death .
Metabolic Pathways
This compound is involved in several metabolic pathways, including hydrolysis, oxidation, hydrogenation, dehydration, and combination with sulfate . These metabolic processes are facilitated by enzymes such as cytochrome P450s, which play a crucial role in the biotransformation of this compound. The compound’s metabolism affects metabolic flux and metabolite levels, influencing its overall pharmacological activity.
Transport and Distribution
Within cells and tissues, this compound is transported and distributed through various mechanisms. The compound interacts with transporters and binding proteins that facilitate its localization and accumulation in specific cellular compartments . These interactions are essential for the compound’s bioavailability and therapeutic efficacy. This compound’s distribution within tissues also determines its cytotoxic effects on cancer cells.
Subcellular Localization
This compound’s subcellular localization plays a critical role in its activity and function. The compound is primarily localized in the mitochondria, where it induces apoptosis by disrupting mitochondrial membrane potential and releasing cytochrome c . Additionally, this compound may undergo post-translational modifications that direct it to specific organelles, enhancing its therapeutic effects.
Preparation Methods
Synthetic Routes and Reaction Conditions
The synthesis of Scutebarbatine A involves several steps, starting from readily available precursors. The key steps include cyclization, oxidation, and functional group modifications to achieve the desired clerodane diterpenoid structure. Specific reaction conditions, such as temperature, solvents, and catalysts, are optimized to maximize yield and purity .
Industrial Production Methods
Industrial production of this compound is still in the research phase, with efforts focused on optimizing extraction methods from Scutellaria barbata. Traditional Chinese Medicine practices involve boiling the herb in water for two hours, followed by drying the extract to produce a powder . Advances in biotechnology are exploring the use of genetically engineered microorganisms, such as yeast, to produce this compound more sustainably and efficiently .
Chemical Reactions Analysis
Types of Reactions
Scutebarbatine A undergoes various chemical reactions, including:
Oxidation: Introduction of oxygen atoms into the molecule, often using reagents like hydrogen peroxide or potassium permanganate.
Reduction: Removal of oxygen atoms or addition of hydrogen atoms, typically using reagents like sodium borohydride or lithium aluminum hydride.
Substitution: Replacement of one functional group with another, using reagents like halogens or nucleophiles.
Common Reagents and Conditions
Oxidation: Hydrogen peroxide, potassium permanganate, and chromium trioxide.
Reduction: Sodium borohydride, lithium aluminum hydride, and catalytic hydrogenation.
Substitution: Halogens (chlorine, bromine), nucleophiles (amines, thiols), and bases (sodium hydroxide, potassium tert-butoxide).
Major Products
The major products formed from these reactions include various oxidized, reduced, and substituted derivatives of this compound, which can be further studied for their biological activities .
Scientific Research Applications
Scutebarbatine A has a wide range of scientific research applications:
Chemistry: Used as a lead compound for the synthesis of novel diterpenoids with potential therapeutic properties.
Biology: Studied for its role in plant defense mechanisms and its interactions with other plant metabolites.
Medicine: Exhibits significant antitumor activity, particularly against lung carcinoma cells, by inducing apoptosis through mitochondrial pathways.
Comparison with Similar Compounds
Similar Compounds
- Scutebarbatine B
- Scutebarbatine C
- Barbatins C
- Baicalin
- Baicalein
Uniqueness
Scutebarbatine A is unique due to its selective cytotoxicity towards cancer cells, sparing normal cells. This selectivity is attributed to its ability to specifically target and down-regulate IAPs, which are often overexpressed in cancer cells . Additionally, its potent antitumor activity and ability to induce apoptosis through the mitochondrial pathway make it a promising candidate for anticancer drug development .
Biological Activity
Scutebarbatine A (SBT-A), a neo-Clerodane diterpenoid alkaloid derived from Scutellaria barbata, has garnered significant attention in recent years due to its promising biological activities, particularly its antitumor effects. This article provides a comprehensive overview of the biological activity of SBT-A, focusing on its mechanisms of action, efficacy in various cancer cell lines, and metabolic pathways.
SBT-A exhibits substantial antitumor activity through several mechanisms, primarily inducing apoptosis in cancer cells. Research indicates that SBT-A triggers apoptosis via the mitochondrial pathway, characterized by the up-regulation of pro-apoptotic proteins such as cytochrome c and caspases (caspase-3 and -9), alongside the down-regulation of anti-apoptotic proteins like Bcl-2 .
Key Findings:
- Cell Line Studies : In vitro studies on A549 lung cancer cells revealed an IC50 value of 39.21 μg/mL for SBT-A, indicating significant cytotoxicity . Furthermore, morphological assessments showed typical apoptotic features such as nuclear condensation and fragmentation after treatment with SBT-A.
- In Vivo Efficacy : In vivo studies using a nude mouse model demonstrated that SBT-A significantly suppressed tumor growth when administered at a dosage of 40 mg/kg/day .
1.2 Selectivity Against Cancer Cells
SBT-A has shown tumor-selective cytotoxicity. For instance, studies on Caco-2 colon cancer cells indicated that treatment with SBT-A at concentrations as low as 60 µM led to a three-fold increase in late apoptotic cells compared to controls . In contrast, normal colonic epithelial cells (HCoEpiC) exhibited minimal apoptosis under similar conditions, underscoring SBT-A's potential as a targeted anticancer agent .
2. Comparative Biological Activity
The following table summarizes the biological activities of SBT-A across different cancer cell lines:
Cell Line | IC50 Value (µM) | Mechanism | Notes |
---|---|---|---|
A549 (Lung) | 39.21 | Mitochondria-mediated apoptosis | Significant morphological changes observed |
Caco-2 (Colon) | 60 | Apoptosis induction | Selective cytotoxicity against cancer cells |
SKOV3 (Ovary) | Not specified | Not explicitly studied | Potential for ovarian cancer treatment |
3. Metabolic Pathways
Understanding the metabolism of SBT-A is crucial for elucidating its pharmacological mechanisms. A study identified 20 metabolites of SBT-A in rat plasma, including 16 phase I and 4 phase II metabolites . The primary metabolic pathways involved hydrolysis, oxidation, and conjugation with sulfate.
4.1 Clinical Relevance
SBT-A's role in traditional Chinese medicine (TCM) highlights its historical use in treating various cancers. Recent clinical studies have begun to explore its efficacy in patients undergoing chemotherapy, suggesting that SBT-A may enhance treatment outcomes while minimizing side effects .
4.2 Future Directions
Further research is warranted to explore the full therapeutic potential of SBT-A, including:
- Comprehensive clinical trials to assess efficacy and safety in humans.
- Investigations into combination therapies with existing chemotherapeutics.
- Exploration of synergistic effects with other bioactive compounds found in Scutellaria barbata.
Properties
IUPAC Name |
[(1R,2S,3R,4R,4aS,8aR)-3-hydroxy-3,4,8,8a-tetramethyl-4-[(E)-2-(5-oxo-2H-furan-3-yl)ethenyl]-2-(pyridine-3-carbonyloxy)-2,4a,5,6-tetrahydro-1H-naphthalen-1-yl] pyridine-3-carboxylate | |
---|---|---|
Source | PubChem | |
URL | https://pubchem.ncbi.nlm.nih.gov | |
Description | Data deposited in or computed by PubChem | |
InChI |
InChI=1S/C32H34N2O7/c1-20-8-5-11-24-30(2,13-12-21-16-25(35)39-19-21)32(4,38)27(41-29(37)23-10-7-15-34-18-23)26(31(20,24)3)40-28(36)22-9-6-14-33-17-22/h6-10,12-18,24,26-27,38H,5,11,19H2,1-4H3/b13-12+/t24-,26+,27+,30-,31+,32+/m1/s1 | |
Source | PubChem | |
URL | https://pubchem.ncbi.nlm.nih.gov | |
Description | Data deposited in or computed by PubChem | |
InChI Key |
CFCKNUOZCOKYOO-JUJIBZTHSA-N | |
Source | PubChem | |
URL | https://pubchem.ncbi.nlm.nih.gov | |
Description | Data deposited in or computed by PubChem | |
Canonical SMILES |
CC1=CCCC2C1(C(C(C(C2(C)C=CC3=CC(=O)OC3)(C)O)OC(=O)C4=CN=CC=C4)OC(=O)C5=CN=CC=C5)C | |
Source | PubChem | |
URL | https://pubchem.ncbi.nlm.nih.gov | |
Description | Data deposited in or computed by PubChem | |
Isomeric SMILES |
CC1=CCC[C@H]2[C@]1([C@H]([C@@H]([C@]([C@]2(C)/C=C/C3=CC(=O)OC3)(C)O)OC(=O)C4=CN=CC=C4)OC(=O)C5=CN=CC=C5)C | |
Source | PubChem | |
URL | https://pubchem.ncbi.nlm.nih.gov | |
Description | Data deposited in or computed by PubChem | |
Molecular Formula |
C32H34N2O7 | |
Source | PubChem | |
URL | https://pubchem.ncbi.nlm.nih.gov | |
Description | Data deposited in or computed by PubChem | |
Molecular Weight |
558.6 g/mol | |
Source | PubChem | |
URL | https://pubchem.ncbi.nlm.nih.gov | |
Description | Data deposited in or computed by PubChem | |
Retrosynthesis Analysis
AI-Powered Synthesis Planning: Our tool employs the Template_relevance Pistachio, Template_relevance Bkms_metabolic, Template_relevance Pistachio_ringbreaker, Template_relevance Reaxys, Template_relevance Reaxys_biocatalysis model, leveraging a vast database of chemical reactions to predict feasible synthetic routes.
One-Step Synthesis Focus: Specifically designed for one-step synthesis, it provides concise and direct routes for your target compounds, streamlining the synthesis process.
Accurate Predictions: Utilizing the extensive PISTACHIO, BKMS_METABOLIC, PISTACHIO_RINGBREAKER, REAXYS, REAXYS_BIOCATALYSIS database, our tool offers high-accuracy predictions, reflecting the latest in chemical research and data.
Strategy Settings
Precursor scoring | Relevance Heuristic |
---|---|
Min. plausibility | 0.01 |
Model | Template_relevance |
Template Set | Pistachio/Bkms_metabolic/Pistachio_ringbreaker/Reaxys/Reaxys_biocatalysis |
Top-N result to add to graph | 6 |
Feasible Synthetic Routes
Disclaimer and Information on In-Vitro Research Products
Please be aware that all articles and product information presented on BenchChem are intended solely for informational purposes. The products available for purchase on BenchChem are specifically designed for in-vitro studies, which are conducted outside of living organisms. In-vitro studies, derived from the Latin term "in glass," involve experiments performed in controlled laboratory settings using cells or tissues. It is important to note that these products are not categorized as medicines or drugs, and they have not received approval from the FDA for the prevention, treatment, or cure of any medical condition, ailment, or disease. We must emphasize that any form of bodily introduction of these products into humans or animals is strictly prohibited by law. It is essential to adhere to these guidelines to ensure compliance with legal and ethical standards in research and experimentation.