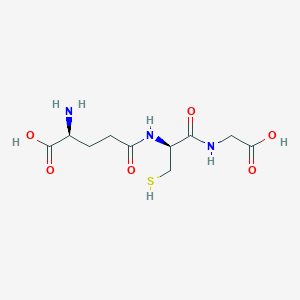
Glycine,N-(N-L-gamma-glutamyl-D-cysteinyl)-
Overview
Description
Glycine, N-(N-L-γ-glutamyl-D-cysteinyl)- is a tripeptide derivative characterized by a γ-glutamyl linkage between L-glutamic acid and D-cysteine, followed by glycine. This structural arrangement distinguishes it from canonical glutathione (γ-glutamyl-L-cysteinylglycine), where L-cysteine is present instead of the D-isomer.
Preparation Methods
Synthetic Routes and Reaction Conditions
Glycine,N-(N-L-gamma-glutamyl-D-cysteinyl)- is synthesized from L-glutamic acid and L-cysteine in the cytoplasm of virtually all cells in an adenosine triphosphate (ATP)-requiring reaction catalyzed by the enzyme glutamate-cysteine ligase . This reaction is the rate-limiting step in the biosynthesis of glutathione .
Industrial Production Methods
Industrial production of this compound typically involves fermentation processes using microorganisms such as Saccharomyces cerevisiae. The production process can be optimized by adjusting the medium composition, including amino acids and other nutrients, and by employing genetic engineering techniques to enhance the yield .
Chemical Reactions Analysis
Types of Reactions
Glycine,N-(N-L-gamma-glutamyl-D-cysteinyl)- undergoes various chemical reactions, including oxidation, reduction, and conjugation reactions. It is a cofactor in multiple enzymatic reactions, such as those catalyzed by glutathione S-transferase enzymes .
Common Reagents and Conditions
Common reagents used in reactions involving this compound include oxidizing agents like hydrogen peroxide and reducing agents such as dithiothreitol. The reactions often occur under physiological conditions, with pH and temperature optimized for enzyme activity .
Major Products
The major products formed from these reactions include oxidized forms of the compound, such as glutathione disulfide, and conjugates with various endogenous and exogenous substances .
Scientific Research Applications
Biochemical Significance
Glycine, N-(N-L-gamma-glutamyl-D-cysteinyl)- is structurally related to glutathione, a crucial antioxidant in biological systems. This compound plays a pivotal role in cellular defense against oxidative stress and is involved in the synthesis of proteins and peptides that are vital for cellular function.
Antioxidant Properties
The compound exhibits antioxidant properties similar to those of glutathione, which is essential for protecting cells from oxidative damage. Research indicates that derivatives of this compound can enhance the cellular antioxidant capacity, thus providing therapeutic benefits in conditions characterized by oxidative stress .
Neuropharmacology
Recent studies have explored the effects of compounds related to glycine on neurotransmitter receptors, particularly N-methyl-D-aspartate (NMDA) receptors. For instance, gamma-L-glutamyl-L-glutamate (a related compound) has been shown to partially activate NMDA receptors and enhance synaptic transmission in neuronal cultures . This suggests potential applications in treating neurological disorders where NMDA receptor modulation is beneficial.
Cancer Research
Glycine and its derivatives have been investigated for their roles in cancer therapy. A study indicated that glycine levels correlate with the progression of multiple myeloma (MM). Elevated glycine concentrations were found in the bone marrow microenvironment of MM patients, suggesting that targeting glycine metabolism could be a therapeutic strategy to inhibit tumor growth . In vivo experiments demonstrated that glycine deprivation enhanced the efficacy of bortezomib, a common treatment for MM, highlighting its potential as a co-therapeutic agent .
Neuroprotective Effects
A study focusing on neuroprotective agents found that compounds similar to glycine, when administered to neuronal cultures, significantly reduced neuronal death induced by oxidative stress. The protective mechanism was attributed to enhanced glutathione synthesis and reduced reactive oxygen species (ROS) levels .
Glycine in Cancer Therapy
In an experimental model using mice with induced multiple myeloma, researchers observed that those on a glycine-free diet showed reduced tumor burden compared to control groups. This finding supports the hypothesis that glycine metabolism plays a critical role in tumor progression and could be targeted for therapeutic benefit .
Data Table: Summary of Applications
Mechanism of Action
The mechanism by which Glycine,N-(N-L-gamma-glutamyl-D-cysteinyl)- exerts its effects involves its role as a precursor to glutathione. It participates in the detoxification of reactive oxygen species and maintains cellular redox balance. The compound activates the Kelch-like ECH-associated protein 1 (Keap1)-Nuclear factor erythroid 2-related factor 2 (Nrf2)-antioxidant response element (ARE) pathway, which regulates the expression of genes involved in antioxidant defense .
Comparison with Similar Compounds
Structural Analogues
Table 1: Key Structural and Chemical Properties
*Calculated based on formula C₁₀H₁₇N₃O₆S.
Functional Comparisons
Redox Activity :
Glutathione’s L-cysteine thiol group is critical for reducing reactive oxygen species (ROS). The D-cysteinyl variant in Glycine, N-(N-L-γ-glutamyl-D-cysteinyl)- may exhibit reduced enzymatic recognition, limiting its participation in glutathione peroxidase/peroxiredoxin pathways .- Stereochemical Impact: D-amino acids are rare in eukaryotes and often resist protease degradation. The D-cysteinyl residue in Glycine, N-(N-L-γ-glutamyl-D-cysteinyl)- may confer metabolic stability but reduce binding affinity to glutathione-S-transferases (GSTs) .
Derivative Applications :
- S-Octylglutathione (GTY) : Hydrophobic modifications enable cell membrane penetration, making it useful for inhibiting GSTs in cancer research .
- Chloroethenyl Derivative (CAS 96614-59-4) : Exhibits mutagenic properties via unscheduled DNA synthesis inhibition, highlighting how substituents alter toxicity profiles .
Biological Activity
Glycine, N-(N-L-gamma-glutamyl-D-cysteinyl)-, also known as a derivative of glutathione, is a tripeptide that plays a significant role in various biological processes. This article explores its biological activities, focusing on its enzymatic interactions, antioxidant properties, and implications in health and disease.
Structure and Composition
The compound is composed of three amino acids: glycine, gamma-glutamic acid, and cysteine. The presence of the gamma-glutamyl moiety is crucial for its biological activity, particularly in interactions with gamma-glutamyl transpeptidase (GGT), an enzyme that catalyzes the cleavage of glutathione and related compounds.
Biological Functions
-
Antioxidant Activity :
Glycine, N-(N-L-gamma-glutamyl-D-cysteinyl)- exhibits significant antioxidant properties. It contributes to the cellular defense against reactive oxygen species (ROS) by participating in the synthesis of glutathione (GSH), a major antioxidant in mammalian cells. GSH plays a pivotal role in maintaining redox homeostasis and protecting cells from oxidative stress . -
Enzymatic Interactions :
- Gamma-Glutamyl Transpeptidase (GGT) : This enzyme is responsible for the hydrolysis of glutathione into its constituent amino acids. Glycine, N-(N-L-gamma-glutamyl-D-cysteinyl)- acts as a substrate for GGT, facilitating the release of cysteine and glycine from extracellular glutathione .
- Cysteine Availability : The cleavage of glutathione by GGT is essential for cysteine availability, which is critical for various metabolic processes including protein synthesis and detoxification mechanisms .
-
Role in Disease :
- Cysteine Deficiency : In GGT-deficient models, such as knockout mice studies, there is a significant increase in urinary glutathione levels and subsequent cysteine deficiency leading to growth retardation and other metabolic disorders . This underscores the importance of Glycine, N-(N-L-gamma-glutamyl-D-cysteinyl)- in maintaining adequate levels of cysteine.
- Cancer Research : Inhibitors of GGT have been explored as potential therapeutic agents in cancer treatment. By limiting cysteine supply to tumors, these inhibitors can potentially reduce tumor growth by impairing the tumor's ability to maintain high intracellular levels of glutathione .
Case Study 1: GGT Knockout Mice
A study on GGT knockout mice revealed that the absence of this enzyme led to a 2400-fold increase in urinary glutathione levels compared to wild-type mice. These knockout mice exhibited severe growth retardation and died within 10 weeks due to cysteine deficiency. This highlights the critical role of Glycine, N-(N-L-gamma-glutamyl-D-cysteinyl)- in metabolic pathways involving cysteine .
Case Study 2: Inhibition of GGT
Research has demonstrated that compounds inhibiting GGT can lower intracellular cysteine concentrations in tumors. For instance, inhibitors such as acivicin were shown to have a significant impact on tumor cell lines by reducing their capacity to synthesize glutathione, thereby enhancing the efficacy of chemotherapeutic agents .
Table 1: Comparison of Antioxidant Activities
Compound | IC50 (µM) | Mechanism |
---|---|---|
Glycine, N-(N-L-gamma-glutamyl-D-cysteinyl)- | 12 | Reduces ROS via GSH synthesis |
Acivicin | 0.81 | Inhibits GGT activity |
L-Glutathione | 5 | Direct scavenger of ROS |
Q & A
Basic Research Questions
Q. What are the recommended methodologies for synthesizing Glycine,N-(N-L-γ-glutamyl-D-cysteinyl)- in a laboratory setting?
Synthesis of this compound typically involves solid-phase peptide synthesis (SPPS) or enzymatic approaches. For chemical synthesis, SPPS using Fmoc/t-Bu protection strategies is preferred to ensure stereochemical fidelity. The D-cysteinyl residue introduces challenges due to its non-natural configuration, requiring careful coupling and deprotection steps to avoid racemization . Enzymatic methods leveraging γ-glutamyltransferases or glutathione synthetase analogs may also be explored, though substrate specificity for D-cysteine derivatives must be validated via kinetic assays . Post-synthesis, purity should be verified using reverse-phase HPLC (≥95% purity, as in ) and mass spectrometry (e.g., MALDI-TOF) .
Q. How can researchers structurally characterize Glycine,N-(N-L-γ-glutamyl-D-cysteinyl)- to confirm its configuration and purity?
A combination of techniques is essential:
- Nuclear Magnetic Resonance (NMR) : 1H/13C NMR to resolve stereochemistry, focusing on the γ-glutamyl and D-cysteinyl moieties. Compare chemical shifts with L-cysteine analogs to confirm D-configuration .
- Mass Spectrometry (MS) : High-resolution MS (e.g., ESI-Q-TOF) to confirm molecular weight (e.g., C12H20N3O6S, MW ~342.37 g/mol) and detect impurities .
- Circular Dichroism (CD) : To assess chiral integrity, particularly for distinguishing D/L-cysteinyl configurations in aqueous solutions .
Q. What analytical methods are optimal for detecting and quantifying this compound in biological matrices?
- Reverse-Phase HPLC : Use C18 columns with UV detection at 214 nm (peptide bond absorption). Mobile phases often include trifluoroacetic acid (TFA) or formic acid to enhance resolution .
- Liquid Chromatography-Tandem MS (LC-MS/MS) : For trace quantification in tissues or fluids, employ multiple reaction monitoring (MRM) transitions specific to the compound’s fragmentation pattern .
- Enzymatic Assays : Customize γ-glutamyltransferase-coupled assays to measure enzymatic cleavage rates, though cross-reactivity with L-cysteine derivatives must be ruled out .
Advanced Research Questions
Q. How does the D-cysteinyl configuration influence the compound’s biochemical activity compared to its L-isomer?
The D-cysteinyl residue alters substrate recognition in enzymatic systems. For example:
- Glutathione-Related Pathways : Unlike the L-isomer (a glutathione precursor), the D-configuration may resist degradation by γ-glutamyl transpeptidases, leading to accumulation in cells. Comparative studies using LC-MS in model organisms (e.g., E. coli) can reveal metabolic stability .
- Toxicity Profiling : shows that structural analogs with dichloroethenyl groups exhibit mutagenicity via unscheduled DNA synthesis (UDS) assays. Apply similar in vitro tests (e.g., mammalian kidney cell lines) to evaluate the D-cysteinyl variant’s genotoxic potential .
Q. What experimental strategies resolve contradictions in reported biological activities of this compound?
- Orthogonal Assays : If cell-based studies conflict with in vitro data, combine biochemical assays (e.g., enzyme inhibition kinetics) with structural analysis (X-ray crystallography or NMR) to identify binding modes .
- Isomer-Specific Probes : Synthesize fluorescent or isotopic labels (e.g., 13C-D-cysteinyl) to track uptake and localization, distinguishing artifacts from true biological interactions .
- Meta-Analysis : Cross-reference toxicity data (e.g., RTECS entries) with recent studies to identify confounding factors like impurities or assay conditions .
Q. How can researchers investigate the compound’s role in redox signaling or metal chelation?
- Electrochemical Profiling : Use cyclic voltammetry to measure thiol-disulfide redox potentials, comparing D- and L-cysteinyl variants. The D-configuration may alter electron transfer kinetics .
- Metal-Binding Studies : Employ isothermal titration calorimetry (ITC) or UV-Vis spectroscopy with transition metals (e.g., Zn²⁺, Cu²⁺) to quantify chelation efficiency. Compare with glutathione to assess competitive binding .
- In Silico Modeling : Molecular dynamics simulations (e.g., AMBER force fields) to predict interactions with redox-sensitive proteins like thioredoxin or metallothioneins .
Q. Methodological Notes
- Stereochemical Validation : Always confirm D/L configurations via CD or chiral HPLC to avoid misinterpretation of bioactivity data .
- Toxicity Screening : Prioritize UDS assays (as in ) for mutagenicity, supplemented by Ames tests for bacterial mutagenesis .
- Data Reproducibility : Document batch-specific purity (e.g., CoA certificates in ) and storage conditions (-20°C for long-term stability) to ensure consistency .
Properties
IUPAC Name |
(2S)-2-amino-5-[[(2S)-1-(carboxymethylamino)-1-oxo-3-sulfanylpropan-2-yl]amino]-5-oxopentanoic acid | |
---|---|---|
Source | PubChem | |
URL | https://pubchem.ncbi.nlm.nih.gov | |
Description | Data deposited in or computed by PubChem | |
InChI |
InChI=1S/C10H17N3O6S/c11-5(10(18)19)1-2-7(14)13-6(4-20)9(17)12-3-8(15)16/h5-6,20H,1-4,11H2,(H,12,17)(H,13,14)(H,15,16)(H,18,19)/t5-,6+/m0/s1 | |
Source | PubChem | |
URL | https://pubchem.ncbi.nlm.nih.gov | |
Description | Data deposited in or computed by PubChem | |
InChI Key |
RWSXRVCMGQZWBV-NTSWFWBYSA-N | |
Source | PubChem | |
URL | https://pubchem.ncbi.nlm.nih.gov | |
Description | Data deposited in or computed by PubChem | |
Canonical SMILES |
C(CC(=O)NC(CS)C(=O)NCC(=O)O)C(C(=O)O)N | |
Source | PubChem | |
URL | https://pubchem.ncbi.nlm.nih.gov | |
Description | Data deposited in or computed by PubChem | |
Isomeric SMILES |
C(CC(=O)N[C@H](CS)C(=O)NCC(=O)O)[C@@H](C(=O)O)N | |
Source | PubChem | |
URL | https://pubchem.ncbi.nlm.nih.gov | |
Description | Data deposited in or computed by PubChem | |
Molecular Formula |
C10H17N3O6S | |
Source | PubChem | |
URL | https://pubchem.ncbi.nlm.nih.gov | |
Description | Data deposited in or computed by PubChem | |
DSSTOX Substance ID |
DTXSID301303707 | |
Record name | L-γ-Glutamyl-D-cysteinylglycine | |
Source | EPA DSSTox | |
URL | https://comptox.epa.gov/dashboard/DTXSID301303707 | |
Description | DSSTox provides a high quality public chemistry resource for supporting improved predictive toxicology. | |
Molecular Weight |
307.33 g/mol | |
Source | PubChem | |
URL | https://pubchem.ncbi.nlm.nih.gov | |
Description | Data deposited in or computed by PubChem | |
CAS No. |
128960-77-0 | |
Record name | L-γ-Glutamyl-D-cysteinylglycine | |
Source | CAS Common Chemistry | |
URL | https://commonchemistry.cas.org/detail?cas_rn=128960-77-0 | |
Description | CAS Common Chemistry is an open community resource for accessing chemical information. Nearly 500,000 chemical substances from CAS REGISTRY cover areas of community interest, including common and frequently regulated chemicals, and those relevant to high school and undergraduate chemistry classes. This chemical information, curated by our expert scientists, is provided in alignment with our mission as a division of the American Chemical Society. | |
Explanation | The data from CAS Common Chemistry is provided under a CC-BY-NC 4.0 license, unless otherwise stated. | |
Record name | L-γ-Glutamyl-D-cysteinylglycine | |
Source | EPA DSSTox | |
URL | https://comptox.epa.gov/dashboard/DTXSID301303707 | |
Description | DSSTox provides a high quality public chemistry resource for supporting improved predictive toxicology. | |
Disclaimer and Information on In-Vitro Research Products
Please be aware that all articles and product information presented on BenchChem are intended solely for informational purposes. The products available for purchase on BenchChem are specifically designed for in-vitro studies, which are conducted outside of living organisms. In-vitro studies, derived from the Latin term "in glass," involve experiments performed in controlled laboratory settings using cells or tissues. It is important to note that these products are not categorized as medicines or drugs, and they have not received approval from the FDA for the prevention, treatment, or cure of any medical condition, ailment, or disease. We must emphasize that any form of bodily introduction of these products into humans or animals is strictly prohibited by law. It is essential to adhere to these guidelines to ensure compliance with legal and ethical standards in research and experimentation.