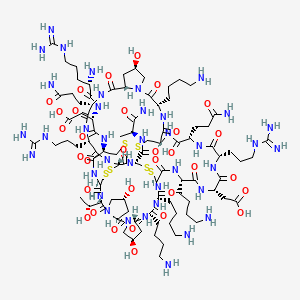
Geographutoxin I
Overview
Description
Geographutoxin I, also known as Mu-conotoxin GIIIA, is a peptide neurotoxin isolated from the marine snail Conus geographus . It consists of 22 amino acid residues including six half-Cys and a C-terminal amide . This toxin potently blocks rat Nav1.4/SCN4A and moderately blocks rNav1.1/SCN1A, rNav1.2/SCN2A, and mNav1.6/SCN8A .
Synthesis Analysis
The synthesis of Geographutoxin I involves the formation of three intramolecular disulfide linkages . These linkages were found to be between Cysr and Cysis, Cy@ and Cys?, and Cys’O and Cys2i . This indicates that Geographutoxin I has a rigid conformation consisting of three loops stabilized by these three disulfide linkages .Molecular Structure Analysis
The molecular structure of Geographutoxin I is characterized by its three intramolecular disulfide linkages . These linkages contribute to the formation of a rigid conformation consisting of three loops . The amino acid sequence of Geographutoxin I was modified by replacing each residue with Ala or Lys to elucidate its active center for blocking sodium channels of skeletal muscle .Chemical Reactions Analysis
The chemical reactions involving Geographutoxin I primarily concern its interaction with sodium channels. The toxin potently blocks rat Nav1.4/SCN4A and moderately blocks rNav1.1/SCN1A, rNav1.2/SCN2A, and mNav1.6/SCN8A .Scientific Research Applications
Neurological Research
Geographutoxin I, derived from the marine snail Conus geographus, is a peptide toxin . It has been found to have inhibitory effects on the contractile response of the mouse diaphragm to direct stimulation . This makes it a valuable tool in neurological research, particularly in studying the mechanisms of neuromuscular transmission.
Mechanism of Action
Result of Action
The primary result of Geographutoxin I’s action is the inhibition of muscle contraction . By blocking the sodium channels, the toxin prevents the propagation of action potentials in the muscle cells, leading to a loss of muscle contractility . This can result in paralysis in the affected muscles .
Safety and Hazards
properties
IUPAC Name |
(3S)-3-[[(2S)-2-amino-5-carbamimidamidopentanoyl]amino]-4-oxo-4-[[(1R,4S,7S,10S,12R,16S,19R,22S,25S,28S,31S,34R,37S,40S,43S,45R,49S,51R,55S,58R,65R,72R)-16,31,37,40-tetrakis(4-aminobutyl)-65-[[(2S)-1-amino-1-oxopropan-2-yl]carbamoyl]-7,22-bis(3-amino-3-oxopropyl)-4,25-bis(3-carbamimidamidopropyl)-28-(carboxymethyl)-12,45,51-trihydroxy-55-[(1R)-1-hydroxyethyl]-3,6,9,15,18,21,24,27,30,33,36,39,42,48,54,57,63,71-octadecaoxo-60,61,67,68,74,75-hexathia-2,5,8,14,17,20,23,26,29,32,35,38,41,47,53,56,64,70-octadecazahexacyclo[32.28.7.719,58.010,14.043,47.049,53]hexaheptacontan-72-yl]amino]butanoic acid | |
---|---|---|
Source | PubChem | |
URL | https://pubchem.ncbi.nlm.nih.gov | |
Description | Data deposited in or computed by PubChem | |
InChI |
InChI=1S/C100H170N38O32S6/c1-46(76(108)149)118-87(160)62-40-171-172-41-63-88(161)122-52(15-3-7-25-101)80(153)128-61(36-74(147)148)85(158)121-55(19-12-30-116-99(111)112)79(152)123-57(21-23-71(106)143)84(157)131-64-42-173-175-44-66(132-86(159)60(35-73(145)146)127-77(150)51(105)14-11-29-115-98(109)110)91(164)134-67(92(165)135-75(47(2)139)97(170)138-39-50(142)34-70(138)96(169)137-38-49(141)33-69(137)94(167)124-54(17-5-9-27-103)78(151)119-53(81(154)129-63)16-4-8-26-102)45-176-174-43-65(90(163)133-62)130-82(155)56(20-13-31-117-100(113)114)120-83(156)58(22-24-72(107)144)125-93(166)68-32-48(140)37-136(68)95(168)59(126-89(64)162)18-6-10-28-104/h46-70,75,139-142H,3-45,101-105H2,1-2H3,(H2,106,143)(H2,107,144)(H2,108,149)(H,118,160)(H,119,151)(H,120,156)(H,121,158)(H,122,161)(H,123,152)(H,124,167)(H,125,166)(H,126,162)(H,127,150)(H,128,153)(H,129,154)(H,130,155)(H,131,157)(H,132,159)(H,133,163)(H,134,164)(H,135,165)(H,145,146)(H,147,148)(H4,109,110,115)(H4,111,112,116)(H4,113,114,117)/t46-,47+,48+,49+,50+,51-,52-,53-,54-,55-,56-,57-,58-,59-,60-,61-,62-,63-,64-,65-,66-,67-,68-,69-,70-,75-/m0/s1 | |
Source | PubChem | |
URL | https://pubchem.ncbi.nlm.nih.gov | |
Description | Data deposited in or computed by PubChem | |
InChI Key |
JXBJHMUQZOSJPJ-HTVVLJMASA-N | |
Source | PubChem | |
URL | https://pubchem.ncbi.nlm.nih.gov | |
Description | Data deposited in or computed by PubChem | |
Canonical SMILES |
CC(C1C(=O)N2CC(CC2C(=O)N3CC(CC3C(=O)NC(C(=O)NC(C(=O)NC4CSSCC(NC(=O)C5CSSCC(C(=O)N1)NC(=O)C(CSSCC(C(=O)NC(C(=O)N6CC(CC6C(=O)NC(C(=O)NC(C(=O)N5)CCCNC(=N)N)CCC(=O)N)O)CCCCN)NC(=O)C(NC(=O)C(NC(=O)C(NC(=O)C(NC4=O)CCCCN)CC(=O)O)CCCNC(=N)N)CCC(=O)N)NC(=O)C(CC(=O)O)NC(=O)C(CCCNC(=N)N)N)C(=O)NC(C)C(=O)N)CCCCN)CCCCN)O)O)O | |
Source | PubChem | |
URL | https://pubchem.ncbi.nlm.nih.gov | |
Description | Data deposited in or computed by PubChem | |
Isomeric SMILES |
C[C@H]([C@H]1C(=O)N2C[C@@H](C[C@H]2C(=O)N3C[C@@H](C[C@H]3C(=O)N[C@H](C(=O)N[C@H](C(=O)N[C@H]4CSSC[C@H](NC(=O)[C@@H]5CSSC[C@@H](C(=O)N1)NC(=O)[C@H](CSSC[C@@H](C(=O)N[C@H](C(=O)N6C[C@@H](C[C@H]6C(=O)N[C@H](C(=O)N[C@H](C(=O)N5)CCCNC(=N)N)CCC(=O)N)O)CCCCN)NC(=O)[C@@H](NC(=O)[C@@H](NC(=O)[C@@H](NC(=O)[C@@H](NC4=O)CCCCN)CC(=O)O)CCCNC(=N)N)CCC(=O)N)NC(=O)[C@H](CC(=O)O)NC(=O)[C@H](CCCNC(=N)N)N)C(=O)N[C@@H](C)C(=O)N)CCCCN)CCCCN)O)O)O | |
Source | PubChem | |
URL | https://pubchem.ncbi.nlm.nih.gov | |
Description | Data deposited in or computed by PubChem | |
Molecular Formula |
C100H170N38O32S6 | |
Source | PubChem | |
URL | https://pubchem.ncbi.nlm.nih.gov | |
Description | Data deposited in or computed by PubChem | |
DSSTOX Substance ID |
DTXSID101339126 | |
Record name | mu-Conotoxin G IIIA | |
Source | EPA DSSTox | |
URL | https://comptox.epa.gov/dashboard/DTXSID101339126 | |
Description | DSSTox provides a high quality public chemistry resource for supporting improved predictive toxicology. | |
Molecular Weight |
2609.1 g/mol | |
Source | PubChem | |
URL | https://pubchem.ncbi.nlm.nih.gov | |
Description | Data deposited in or computed by PubChem | |
Product Name |
Geographutoxin I | |
CAS RN |
86394-16-3, 129129-65-3 | |
Record name | Geographutoxin I | |
Source | ChemIDplus | |
URL | https://pubchem.ncbi.nlm.nih.gov/substance/?source=chemidplus&sourceid=0086394163 | |
Description | ChemIDplus is a free, web search system that provides access to the structure and nomenclature authority files used for the identification of chemical substances cited in National Library of Medicine (NLM) databases, including the TOXNET system. | |
Record name | mu-Conotoxin G IIIA | |
Source | ChemIDplus | |
URL | https://pubchem.ncbi.nlm.nih.gov/substance/?source=chemidplus&sourceid=0129129653 | |
Description | ChemIDplus is a free, web search system that provides access to the structure and nomenclature authority files used for the identification of chemical substances cited in National Library of Medicine (NLM) databases, including the TOXNET system. | |
Record name | mu-Conotoxin G IIIA | |
Source | EPA DSSTox | |
URL | https://comptox.epa.gov/dashboard/DTXSID101339126 | |
Description | DSSTox provides a high quality public chemistry resource for supporting improved predictive toxicology. | |
Record name | 129129-65-3 | |
Source | European Chemicals Agency (ECHA) | |
URL | https://echa.europa.eu/information-on-chemicals | |
Description | The European Chemicals Agency (ECHA) is an agency of the European Union which is the driving force among regulatory authorities in implementing the EU's groundbreaking chemicals legislation for the benefit of human health and the environment as well as for innovation and competitiveness. | |
Explanation | Use of the information, documents and data from the ECHA website is subject to the terms and conditions of this Legal Notice, and subject to other binding limitations provided for under applicable law, the information, documents and data made available on the ECHA website may be reproduced, distributed and/or used, totally or in part, for non-commercial purposes provided that ECHA is acknowledged as the source: "Source: European Chemicals Agency, http://echa.europa.eu/". Such acknowledgement must be included in each copy of the material. ECHA permits and encourages organisations and individuals to create links to the ECHA website under the following cumulative conditions: Links can only be made to webpages that provide a link to the Legal Notice page. | |
Q & A
Q1: What are the known biological effects of Geographutoxin I?
A1: Geographutoxin I exhibits inhibitory effects on the contractile response of the mouse diaphragm to direct stimulation [, , ]. This suggests that the toxin likely targets components of the neuromuscular system, disrupting signal transmission and muscle contraction.
A2: While the complete three-dimensional structure of Geographutoxin I has not been fully elucidated, research has provided valuable insights. The amino acid composition of the toxin has been determined [, ]. Furthermore, studies have successfully identified the disulfide pairings within the Geographutoxin I peptide []. This structural information is crucial for understanding the toxin's interaction with its biological targets.
Q2: What research tools and resources are valuable for studying Geographutoxin I?
A3: A multidisciplinary approach is crucial for unraveling the complexities of Geographutoxin I. Pharmacological studies using isolated tissues or cells can help pinpoint the toxin's mechanism of action []. Biochemical techniques like chromatography are essential for isolating and purifying the toxin [, ]. Advanced tools like mass spectrometry can contribute to structural characterization. Furthermore, computational chemistry and molecular modeling could offer insights into the toxin's interactions with its targets, but such studies have yet to be published.
Disclaimer and Information on In-Vitro Research Products
Please be aware that all articles and product information presented on BenchChem are intended solely for informational purposes. The products available for purchase on BenchChem are specifically designed for in-vitro studies, which are conducted outside of living organisms. In-vitro studies, derived from the Latin term "in glass," involve experiments performed in controlled laboratory settings using cells or tissues. It is important to note that these products are not categorized as medicines or drugs, and they have not received approval from the FDA for the prevention, treatment, or cure of any medical condition, ailment, or disease. We must emphasize that any form of bodily introduction of these products into humans or animals is strictly prohibited by law. It is essential to adhere to these guidelines to ensure compliance with legal and ethical standards in research and experimentation.