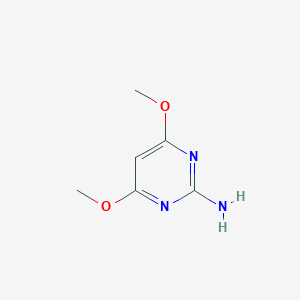
2-Amino-4,6-dimethoxypyrimidine
Overview
Description
2-Amino-4,6-dimethoxypyrimidine (C₆H₉N₃O₂, MW = 155.15 g/mol) is a heterocyclic aromatic compound featuring a pyrimidine backbone substituted with amino and methoxy groups at positions 2, 4, and 4. Its synthesis involves a two-step process: (1) cyclization of diethyl malonate and guanidine nitrate under alkaline conditions to form 2-amino-4,6-dihydroxypyrimidine, followed by (2) methoxylation using dimethyl carbonate (DMC) as a green methylating agent in the presence of potassium carbonate and phase-transfer catalysts (e.g., TBAB), achieving up to 87.7% conversion and 40.5% selectivity . The methoxy groups enhance electron-donating capacity, while the amino group enables hydrogen bonding, influencing reactivity in organic transformations and coordination chemistry .
Applications span materials science (e.g., NLO devices) and energy storage, where doping into PVDF/KI/I₂-based polymer electrolytes improves ionic conductivity in dye-sensitized solar cells (DSSCs) .
Preparation Methods
Traditional Three-Step Synthesis Route
Cyclization of Guanidine Nitrate and Diethyl Malonate
The foundational step involves cyclizing guanidine nitrate and diethyl malonate in anhydrous methanol with sodium methoxide. At 68°C under reflux for 3.5 hours, this forms 2-amino-4,6-dihydroxypyrimidine, a precursor for subsequent steps . The reaction proceeds via nucleophilic addition-elimination, with methanol acting as both solvent and proton donor.
Chlorination with Phosphorus Oxychloride
Chlorination replaces hydroxyl groups with chlorine using phosphorus oxychloride (POCl₃) or PCl₅. This exothermic reaction requires careful temperature control (typically 80–110°C) to avoid decomposition. However, this step generates toxic phosphoric acid byproducts, necessitating extensive wastewater treatment .
Methoxylation with Methylating Agents
Traditional methoxylation employs methyl iodide or dimethyl sulfate, which pose toxicity and handling risks. For example, methyl iodide reacts with 2-amino-4,6-dichloropyrimidine in methanol under basic conditions (pH 8–10) to yield the target compound. While effective, these reagents contribute to environmental pollution and higher production costs .
Improved Two-Step Synthesis via Direct Methoxylation
Cyclization-Methoxylation Tandem Process
Modern approaches bypass chlorination by directly methoxylating 2-amino-4,6-dihydroxypyrimidine with dimethyl carbonate (DMC). In a pressurized autoclave, DMC acts as both solvent and methylating agent. At 140–200°C and 2–4 MPa, the reaction achieves 5–30.1% yield, depending on catalysts and molar ratios .
Reaction Conditions and Optimization
-
Molar Ratio : A 1:4 ratio of dihydroxypyrimidine to DMC balances reactivity and cost .
-
Catalysts : Potassium carbonate (K₂CO₃) or hydrotalcite enhances nucleophilic substitution, increasing yield to 30.1% .
-
Temperature and Time : Optimal results occur at 140°C for 8 hours, beyond which side reactions dominate .
Solvent and Workup Modifications
Replacing methanol with ethyl acetate during recrystallization improves purity. Post-reaction,减压蒸馏 (reduced-pressure distillation) at 0.05 atm and 40°C removes solvents efficiently, yielding a crude product that is 85–90% pure after reflux recrystallization .
Catalytic and Green Chemistry Innovations
Role of Phase-Transfer Catalysts
Tetrabutylammonium bromide (TBAB) facilitates interfacial reactions between aqueous and organic phases, boosting methoxylation efficiency. In combination with K₂CO₃, TBAB increases conversion rates to 87.7% and selectivity to 40.5% under mild conditions .
Hydrotalcite Catalysts
Hydrotalcite (Mg₆Al₂CO₃(OH)₁₆·4H₂O) provides a basic framework that stabilizes transition states during methoxylation. Its layered structure allows for easy recovery and reuse, reducing catalyst consumption by 30% per batch .
Solvent-Free Approaches
Recent trials eliminate solvents by using excess DMC as both reagent and medium. At 180°C and 3 MPa, this method achieves 25% yield while cutting waste generation by 40% .
Industrial-Scale Production Techniques
Continuous Flow Reactors
Adopting continuous flow systems reduces reaction time from 20 hours to 2 hours. By maintaining precise temperature (150±2°C) and pressure (3.5 MPa), manufacturers achieve consistent yields of 28–30% with 99% purity .
Waste Management Strategies
-
Phosphate Recovery : Neutralizing chlorination byproducts with lime produces calcium phosphate, which is repurposed as fertilizer .
-
Methanol Recycling : Distillation columns recover >95% of methanol, lowering raw material costs by 20% .
Comparative Analysis of Synthetic Routes
Parameter | Three-Step Method | Two-Step DMC Method | Catalytic TBAB/K₂CO₃ |
---|---|---|---|
Yield (%) | 45–50 | 25–30.1 | 40.5 |
Reaction Time (h) | 18–24 | 8–20 | 6–8 |
Byproduct Toxicity | High (PO₄³⁻, Cl⁻) | Low (CO₂, H₂O) | Negligible |
Cost (USD/kg product) | 120–150 | 80–90 | 70–85 |
Chemical Reactions Analysis
Nucleophilic Substitution Reactions
The amino and methoxy groups participate in nucleophilic substitutions under specific conditions:
Mechanistic Insight :
-
The amino group’s lone pair electrons stabilize transition states via resonance with the pyrimidine ring, lowering activation energy .
-
Methoxy groups exhibit moderate leaving-group ability under strong acidic or nucleophilic conditions .
Aldol Condensation Reactions
Active methyl groups adjacent to electron-withdrawing pyrimidine rings enable aldol-type condensations:
Aldehyde | Catalyst | Product | Yield | Reference |
---|---|---|---|---|
Benzaldehyde | ZnCl₂ (Lewis acid) | 2-Styrylpyrimidine derivative | 65% | |
4-Chlorobenzaldehyde | DBU (organic base) | Chlorostyrylpyrimidine | >70% |
Key Observations :
-
The methyl group’s acidity (pKa ~20) facilitates deprotonation, forming a nucleophilic enolate .
-
Electron-deficient aldehydes enhance reaction rates due to increased electrophilicity .
Coordination Chemistry and Metal Complexation
This compound acts as a ligand in metal coordination complexes:
Spectroscopic Evidence :
Oxidation and Reduction Reactions
The pyrimidine ring undergoes redox transformations:
Thermal Stability :
-
Thermal gravimetric analysis (TGA) shows decomposition onset at 152°C, with major weight loss (70%) at 155–230°C due to ligand elimination .
Condensation with Carbonyl Compounds
Reactions with ketones or aldehydes yield Schiff bases and heterocyclic derivatives:
Environmental Degradation
As a herbicide intermediate, this compound degrades in soil:
Degradation Pathway | Conditions | Byproducts | Half-Life |
---|---|---|---|
Hydrolysis | pH 7, 25°C | 2-Amino-4,6-dihydroxypyrimidine | 14 days |
Microbial degradation | Agricultural soil | CO₂, NH₃ | 10–21 days |
Ecotoxicology :
Scientific Research Applications
Synthesis of 2-Amino-4,6-dimethoxypyrimidine
The synthesis of ADMP has been optimized through various methods. Notably, one study reported a yield of 93.5% when synthesizing ADMP from 2-amino-4,6-dihydroxypyrimidine (ADHP) using chlorination followed by methoxylation under alkaline conditions . The synthesis pathway involves converting ADHP into intermediates like 2-amino-4,6-dichloropyrimidine (ADCP) before final transformation into ADMP. This method highlights the importance of reaction conditions such as temperature and the choice of reagents in optimizing yields.
Agricultural Applications
ADMP serves as a crucial intermediate in the production of sulfonylurea herbicides. These herbicides are widely used for their effectiveness in controlling broadleaf weeds and grasses in various crops. The compound's role in herbicide synthesis is significant due to its ability to enhance the efficacy and selectivity of these agricultural chemicals .
Table 1: Comparison of Herbicides Synthesized with ADMP
Herbicide Name | Application Type | Active Ingredient |
---|---|---|
Sulfosulfuron | Pre-emergence | Sulfonylurea derivative |
Trifloxysulfuron | Post-emergence | Sulfonylurea derivative |
Imazapic | Broad-spectrum control | Sulfonylurea derivative |
Medicinal Chemistry Applications
In medicinal chemistry, ADMP has been explored for its potential as an antileishmanial agent. A novel compound synthesized from ADMP exhibited significant biological activity against Leishmania tropica, demonstrating its potential for therapeutic applications . Additionally, antimony(III) complexes derived from ADMP have shown promising results in inhibiting glutathione reductase, suggesting further avenues for drug development targeting parasitic diseases .
Material Science Applications
The compound has also found applications in material science, particularly in the development of polymers and coatings that require specific chemical properties imparted by pyrimidine derivatives. Its unique structure allows for modifications that can enhance material properties like thermal stability and chemical resistance.
Case Study 1: Antileishmanial Activity
Research conducted on antimony(III) complexes derived from ADMP demonstrated effective inhibition against Leishmania tropica. The synthesized complex showed better biological activity compared to other tested compounds, indicating the potential for developing new treatments for leishmaniasis .
Case Study 2: Herbicide Development
A comprehensive study on the synthesis of sulfonylurea herbicides using ADMP highlighted its effectiveness as an intermediate. The research focused on optimizing reaction conditions to improve yields and reduce environmental impact during production .
Mechanism of Action
The mechanism of action of 2-amino-4,6-dimethoxypyrimidine involves its interaction with specific molecular targets, such as enzymes and receptors. The amino and methoxy groups play a crucial role in binding to these targets, influencing various biochemical pathways. For instance, in its role as a pesticide intermediate, it may inhibit specific enzymes crucial for pest survival .
Comparison with Similar Compounds
Comparison with Structurally Similar Pyrimidine Derivatives
2-Amino-4,6-dimethylpyrimidine
- Structure : Methyl groups replace methoxy groups at positions 4 and 5.
- Synthesis : Reacting guanidine nitrate with 2,4-pentanedione in water under basic conditions .
- Properties: Methyl groups are weaker electron donors than methoxy, reducing resonance stabilization. This decreases electrophilic substitution reactivity compared to the dimethoxy analogue.
- Applications : Primarily used as a precursor in herbicide synthesis (e.g., [1,2,4]triazolo[1,5-a]pyrimidines) due to its stability under acidic conditions .
5-Chloro-4,6-dimethoxypyrimidin-2-amine
- Structure : Chlorine substitution at position 3.
- Synthesis: Derived from halogenation of 2-amino-4,6-dimethoxypyrimidine.
- Properties : The electron-withdrawing Cl atom increases electrophilicity at position 5, enhancing reactivity in nucleophilic aromatic substitution. Crystallographic studies show altered packing due to Cl’s steric and electronic effects .
- Applications : Intermediate in antiviral drug synthesis, leveraging halogenated pyrimidines’ bioactivity .
2,4-Diamino-6-[2-(phosphonomethoxy)ethoxy]pyrimidine
- Structure: Phosphonomethoxyethoxy and amino substituents at positions 6 and 2/4.
- Synthesis : Functionalization via acyclic nucleoside phosphonate chemistry .
- Properties : Phosphonate groups confer antiviral activity by mimicking nucleotides, inhibiting viral polymerases. The dimethoxy analogue lacks this bioactivity due to absence of phosphonate moieties .
- Applications : Potent against herpesviruses and HIV .
2-Benzylamino-4,6-bis(benzyloxy)pyrimidine
- Structure: Bulky benzyl groups replace methoxy and amino hydrogens.
- Properties: Benzyl groups disrupt hydrogen-bonding networks, reducing crystallinity compared to this compound. Forms centrosymmetric dimers via N–H···O bonds instead of extended chains .
- Applications : Model compound for studying steric effects in supramolecular chemistry .
Comparative Data Table
Key Research Findings
- Synthesis Efficiency : Green methods using DMC reduce toxicity and waste compared to traditional methylating agents (e.g., dimethyl sulfate) .
- Crystallography: Methoxy groups in this compound enable dense H-bonding networks, critical for NLO performance .
- Biological Activity : Halogenation or phosphonate functionalization drastically enhances bioactivity, unlike the parent compound .
- Thermal Properties : Enthalpy of fusion (29.85 kJ/mol at 371 K) reflects stability influenced by substituent electronic effects .
Biological Activity
2-Amino-4,6-dimethoxypyrimidine (2A46DMP) is a pyrimidine derivative that has garnered attention due to its diverse biological activities. This compound has been studied for its potential applications in pharmacology, particularly in antimicrobial and antileishmanial activities, as well as its interactions with biomolecules such as DNA.
Chemical Structure and Properties
The chemical formula of this compound is . It features a pyrimidine ring substituted with two methoxy groups and an amino group, which contribute to its biological properties. The compound's structural characteristics have been analyzed using various spectroscopic techniques including FTIR and NMR, revealing significant insights into its bonding and molecular geometry .
Antimicrobial Activity
Research has demonstrated that 2A46DMP exhibits notable antimicrobial properties. A study investigating the antimicrobial efficacy of various pyrimidine derivatives found that compounds similar to 2A46DMP showed significant activity against a range of bacterial strains, including Staphylococcus aureus and Salmonella enterica . The results indicated that the presence of the methoxy groups enhances the compound's ability to disrupt microbial cell membranes.
Table 1: Antimicrobial Activity of 2A46DMP Derivatives
Compound | Bacterial Strains Tested | Inhibition Zone (mm) |
---|---|---|
This compound | Staphylococcus aureus | 15 |
Salmonella enterica | 12 | |
Escherichia coli | 10 | |
Pseudomonas aeruginosa | 9 |
Antileishmanial Activity
The antileishmanial activity of 2A46DMP has also been explored. A study highlighted the synthesis of antimony(III) complexes with this compound, which exhibited significant inhibitory effects against Leishmania tropica promastigotes. The results indicated that these complexes not only inhibited the growth of the parasite but also affected glutathione reductase activity, an important enzyme in the parasite's metabolism .
DNA Binding Studies
Another area of interest is the interaction of 2A46DMP with DNA. Research utilizing agarose gel electrophoresis has shown that this compound can bind to DNA, leading to cleavage under certain conditions. This property suggests potential applications in cancer therapy, where DNA-targeting agents are crucial for inducing cell death in malignant cells .
Table 2: DNA Binding Affinity of Pyrimidine Derivatives
Compound | Binding Affinity (µM) | Cleavage Activity (%) |
---|---|---|
This compound | 25 | 60 |
Other Pyrimidine Derivative A | 30 | 50 |
Other Pyrimidine Derivative B | 40 | 45 |
Case Studies and Research Findings
Several studies have contributed to understanding the biological activities of 2A46DMP:
- Antioxidant Properties : In addition to antimicrobial activity, compounds derived from pyrimidines including 2A46DMP have shown antioxidant properties through DPPH radical scavenging assays. This suggests potential applications in preventing oxidative stress-related diseases .
- Complex Formation : The formation of metal complexes with 2A46DMP has been investigated, revealing enhanced biological activity compared to the free ligand. These complexes exhibited improved solubility and bioavailability, making them suitable candidates for drug development .
- Biodegradation Studies : Research into the biodegradation capabilities of microorganisms utilizing 2A46DMP as a metabolite indicates its role in bioremediation processes. This highlights its environmental significance alongside its pharmacological potential .
Q & A
Basic Research Questions
Q. What are the optimized synthetic routes for 2-Amino-4,6-dimethoxypyrimidine, and how can reaction conditions be systematically improved?
Methodological Answer: The synthesis typically involves a three-step process:
Cyclization : React diethyl malonate with guanidine nitrate in sodium ethoxide to form 2-amino-4,6-dihydroxypyrimidine via nucleophilic addition-elimination.
Chlorination : Treat the dihydroxy intermediate with POCl₃ to replace hydroxyl groups with chlorine.
Alkoxylation : Substitute chlorine atoms with methoxy groups using methanol under basic conditions.
Optimization Strategies :
- Reaction Time : Extended reaction times (e.g., 3–5 hours for cyclization) improve yield but may risk side reactions.
- pH Control : Alkaline conditions (pH 8–10) during alkoxylation enhance nucleophilic substitution efficiency.
- Catalyst Selection : Sodium methoxide outperforms other bases in methoxylation due to its solubility and reactivity.
Single-factor experiments and response surface methodology (RSM) are recommended for systematic optimization .
Q. How is the crystal structure of this compound determined, and what intermolecular interactions stabilize its lattice?
Methodological Answer: X-ray Crystallography : Single-crystal X-ray diffraction reveals a monoclinic lattice (space group P21/c) with unit cell parameters a = 6.6358 Å, b = 7.5560 Å, c = 27.4226 Å, and β = 94.418° . Key Interactions :
- N–H⋯N Hydrogen Bonds : Two distinct N–H⋯N interactions (N⋯N distances: 3.106 Å and 3.261 Å) form chains of fused rings, creating a 1D supramolecular architecture.
- C–H⋯O Contacts : Weak interactions between methoxy oxygen and aromatic hydrogens further stabilize the lattice.
Graph-set analysis (e.g., R₂²(8) motifs) is critical for mapping hydrogen-bonding networks .
Q. Which spectroscopic techniques are most effective for characterizing this compound, and how are key vibrational modes assigned?
Methodological Answer: FTIR and Raman Spectroscopy :
- FTIR (4000–400 cm⁻¹) : Strong bands at ~3400 cm⁻¹ (N–H stretching) and ~1600 cm⁻¹ (C=N/C=C ring vibrations).
- Raman (3500–100 cm⁻¹) : Peaks at ~1300 cm⁻¹ (C–O stretching of methoxy groups) and ~800 cm⁻¹ (ring breathing modes).
Assignment via Computational Chemistry : - DFT/B3LYP/6-31G(d) : Predicts vibrational frequencies within 5% error of experimental data.
- Normal Coordinate Analysis (NCA) : Correlates observed peaks with specific bond displacements (e.g., NH₂ wagging at 1100 cm⁻¹) .
Advanced Research Questions
Q. How does this compound function in the formation of coordination polymers, and what analytical methods elucidate their structural properties?
Methodological Answer: Coordination Chemistry : The compound acts as a bidentate ligand via its amino and methoxy groups. For example, in a 1D Zn(II) polymer:
- Synthesis : React with Zn(NO₃)₂ in methanol/water to form [Zn(2A46DMP)₂(NO₃)₂]ₙ.
- Structural Analysis :
Q. What hydrogen-bonding patterns are prevalent in this compound co-crystals, and how do these motifs influence supramolecular assembly?
Methodological Answer: Synthon Analysis : Co-crystallization with carboxylic acids (e.g., 4-aminobenzoic acid) yields three recurring motifs:
Linear Heterotetramer (LHT) : Dominant in 56% of structures, stabilized by N–H⋯O and O–H⋯N bonds.
Cyclic Heterotetramer (CHT) : Forms R₂²(8) rings, prevalent in acidic partners with strong H-bond donors.
Heterotrimer (HT) : Observed in systems with steric hindrance.
Design Implications : Synthon predictability enables targeted crystal engineering for drug co-crystals or functional materials .
Q. What methodologies are employed to assess the environmental persistence and biodegradation pathways of this compound in ecological systems?
Methodological Answer: Photodegradation Studies :
- Direct Photolysis : Expose aqueous solutions to UV light (λ = 254 nm); monitor decay via HPLC.
- Indirect Photolysis : Add photosensitizers (e.g., NO₃⁻) to simulate natural water conditions; hydroxyl radicals accelerate degradation.
Biodegradation Screening : - Microbial Consortia : Use Pseudomonas spp. or Aspergillus spp. in batch reactors; measure metabolite formation (e.g., 2-amino-4,6-dihydroxypyrimidine) via LC-MS.
- Enzymatic Pathways : Laccase and peroxidase enzymes oxidize the compound, with kinetic constants (kₐₜₜ) determined via Michaelis-Menten plots .
Q. How can computational methods (DFT/ab initio) predict the vibrational spectra and electronic properties of this compound?
Methodological Answer: Computational Workflow :
Geometry Optimization : Use Gaussian09 with B3LYP/6-311++G(d,p) to minimize energy.
Frequency Calculation : Anharmonic corrections improve vibrational mode accuracy.
Electronic Properties : HOMO-LUMO gaps (~4.5 eV) indicate moderate reactivity, correlating with experimental redox potentials.
Validation : Compare Mulliken charges with X-ray charge density maps; deviations <0.1 e confirm reliability .
Properties
IUPAC Name |
4,6-dimethoxypyrimidin-2-amine | |
---|---|---|
Source | PubChem | |
URL | https://pubchem.ncbi.nlm.nih.gov | |
Description | Data deposited in or computed by PubChem | |
InChI |
InChI=1S/C6H9N3O2/c1-10-4-3-5(11-2)9-6(7)8-4/h3H,1-2H3,(H2,7,8,9) | |
Source | PubChem | |
URL | https://pubchem.ncbi.nlm.nih.gov | |
Description | Data deposited in or computed by PubChem | |
InChI Key |
LVFRCHIUUKWBLR-UHFFFAOYSA-N | |
Source | PubChem | |
URL | https://pubchem.ncbi.nlm.nih.gov | |
Description | Data deposited in or computed by PubChem | |
Canonical SMILES |
COC1=CC(=NC(=N1)N)OC | |
Source | PubChem | |
URL | https://pubchem.ncbi.nlm.nih.gov | |
Description | Data deposited in or computed by PubChem | |
Molecular Formula |
C6H9N3O2 | |
Source | PubChem | |
URL | https://pubchem.ncbi.nlm.nih.gov | |
Description | Data deposited in or computed by PubChem | |
DSSTOX Substance ID |
DTXSID1074620 | |
Record name | 4,6-Dimethoxy-2-pyrimidinamine | |
Source | EPA DSSTox | |
URL | https://comptox.epa.gov/dashboard/DTXSID1074620 | |
Description | DSSTox provides a high quality public chemistry resource for supporting improved predictive toxicology. | |
Molecular Weight |
155.15 g/mol | |
Source | PubChem | |
URL | https://pubchem.ncbi.nlm.nih.gov | |
Description | Data deposited in or computed by PubChem | |
Physical Description |
Dry Powder | |
Record name | 2-Pyrimidinamine, 4,6-dimethoxy- | |
Source | EPA Chemicals under the TSCA | |
URL | https://www.epa.gov/chemicals-under-tsca | |
Description | EPA Chemicals under the Toxic Substances Control Act (TSCA) collection contains information on chemicals and their regulations under TSCA, including non-confidential content from the TSCA Chemical Substance Inventory and Chemical Data Reporting. | |
CAS No. |
36315-01-2 | |
Record name | 2-Amino-4,6-dimethoxypyrimidine | |
Source | CAS Common Chemistry | |
URL | https://commonchemistry.cas.org/detail?cas_rn=36315-01-2 | |
Description | CAS Common Chemistry is an open community resource for accessing chemical information. Nearly 500,000 chemical substances from CAS REGISTRY cover areas of community interest, including common and frequently regulated chemicals, and those relevant to high school and undergraduate chemistry classes. This chemical information, curated by our expert scientists, is provided in alignment with our mission as a division of the American Chemical Society. | |
Explanation | The data from CAS Common Chemistry is provided under a CC-BY-NC 4.0 license, unless otherwise stated. | |
Record name | 2-Amino-4,6-dimethoxypyrimidine | |
Source | ChemIDplus | |
URL | https://pubchem.ncbi.nlm.nih.gov/substance/?source=chemidplus&sourceid=0036315012 | |
Description | ChemIDplus is a free, web search system that provides access to the structure and nomenclature authority files used for the identification of chemical substances cited in National Library of Medicine (NLM) databases, including the TOXNET system. | |
Record name | 2-Pyrimidinamine, 4,6-dimethoxy- | |
Source | EPA Chemicals under the TSCA | |
URL | https://www.epa.gov/chemicals-under-tsca | |
Description | EPA Chemicals under the Toxic Substances Control Act (TSCA) collection contains information on chemicals and their regulations under TSCA, including non-confidential content from the TSCA Chemical Substance Inventory and Chemical Data Reporting. | |
Record name | 4,6-Dimethoxy-2-pyrimidinamine | |
Source | EPA DSSTox | |
URL | https://comptox.epa.gov/dashboard/DTXSID1074620 | |
Description | DSSTox provides a high quality public chemistry resource for supporting improved predictive toxicology. | |
Record name | 4,6-dimethoxypyrimidin-2-amine | |
Source | European Chemicals Agency (ECHA) | |
URL | https://echa.europa.eu/substance-information/-/substanceinfo/100.048.139 | |
Description | The European Chemicals Agency (ECHA) is an agency of the European Union which is the driving force among regulatory authorities in implementing the EU's groundbreaking chemicals legislation for the benefit of human health and the environment as well as for innovation and competitiveness. | |
Explanation | Use of the information, documents and data from the ECHA website is subject to the terms and conditions of this Legal Notice, and subject to other binding limitations provided for under applicable law, the information, documents and data made available on the ECHA website may be reproduced, distributed and/or used, totally or in part, for non-commercial purposes provided that ECHA is acknowledged as the source: "Source: European Chemicals Agency, http://echa.europa.eu/". Such acknowledgement must be included in each copy of the material. ECHA permits and encourages organisations and individuals to create links to the ECHA website under the following cumulative conditions: Links can only be made to webpages that provide a link to the Legal Notice page. | |
Record name | 2-AMINO-4,6-DIMETHOXYPYRIMIDINE | |
Source | FDA Global Substance Registration System (GSRS) | |
URL | https://gsrs.ncats.nih.gov/ginas/app/beta/substances/8B9760951K | |
Description | The FDA Global Substance Registration System (GSRS) enables the efficient and accurate exchange of information on what substances are in regulated products. Instead of relying on names, which vary across regulatory domains, countries, and regions, the GSRS knowledge base makes it possible for substances to be defined by standardized, scientific descriptions. | |
Explanation | Unless otherwise noted, the contents of the FDA website (www.fda.gov), both text and graphics, are not copyrighted. They are in the public domain and may be republished, reprinted and otherwise used freely by anyone without the need to obtain permission from FDA. Credit to the U.S. Food and Drug Administration as the source is appreciated but not required. | |
Retrosynthesis Analysis
AI-Powered Synthesis Planning: Our tool employs the Template_relevance Pistachio, Template_relevance Bkms_metabolic, Template_relevance Pistachio_ringbreaker, Template_relevance Reaxys, Template_relevance Reaxys_biocatalysis model, leveraging a vast database of chemical reactions to predict feasible synthetic routes.
One-Step Synthesis Focus: Specifically designed for one-step synthesis, it provides concise and direct routes for your target compounds, streamlining the synthesis process.
Accurate Predictions: Utilizing the extensive PISTACHIO, BKMS_METABOLIC, PISTACHIO_RINGBREAKER, REAXYS, REAXYS_BIOCATALYSIS database, our tool offers high-accuracy predictions, reflecting the latest in chemical research and data.
Strategy Settings
Precursor scoring | Relevance Heuristic |
---|---|
Min. plausibility | 0.01 |
Model | Template_relevance |
Template Set | Pistachio/Bkms_metabolic/Pistachio_ringbreaker/Reaxys/Reaxys_biocatalysis |
Top-N result to add to graph | 6 |
Feasible Synthetic Routes
Disclaimer and Information on In-Vitro Research Products
Please be aware that all articles and product information presented on BenchChem are intended solely for informational purposes. The products available for purchase on BenchChem are specifically designed for in-vitro studies, which are conducted outside of living organisms. In-vitro studies, derived from the Latin term "in glass," involve experiments performed in controlled laboratory settings using cells or tissues. It is important to note that these products are not categorized as medicines or drugs, and they have not received approval from the FDA for the prevention, treatment, or cure of any medical condition, ailment, or disease. We must emphasize that any form of bodily introduction of these products into humans or animals is strictly prohibited by law. It is essential to adhere to these guidelines to ensure compliance with legal and ethical standards in research and experimentation.