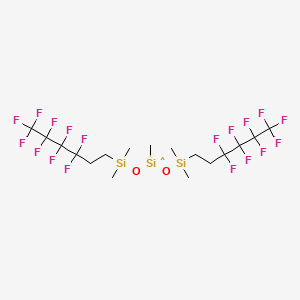
Trisiloxane, 1,1,3,5,5-pentamethyl-1,5-bis(3,3,4,4,5,5,6,6,6-nonafluorohexyl)-
Overview
Description
Trisiloxane, 1,1,3,5,5-pentamethyl-1,5-bis(3,3,4,4,5,5,6,6,6-nonafluorohexyl)- is a specialized organosilicon compound known for its unique chemical properties and applications. This compound is characterized by its siloxane backbone and the presence of fluorinated alkyl groups, which impart distinct hydrophobic and oleophobic properties.
Preparation Methods
Synthetic Routes and Reaction Conditions
The synthesis of Trisiloxane, 1,1,3,5,5-pentamethyl-1,5-bis(3,3,4,4,5,5,6,6,6-nonafluorohexyl)- typically involves the hydrosilylation reaction. This process includes the addition of hydrosilanes to alkenes or alkynes in the presence of a catalyst, often a platinum complex. The reaction conditions usually require a controlled temperature and an inert atmosphere to prevent unwanted side reactions .
Industrial Production Methods
Industrial production of this compound follows similar synthetic routes but on a larger scale. The process involves the use of high-purity reagents and advanced catalytic systems to ensure high yield and purity. The reaction is typically carried out in specialized reactors designed to handle the specific requirements of organosilicon chemistry .
Chemical Reactions Analysis
Types of Reactions
Trisiloxane, 1,1,3,5,5-pentamethyl-1,5-bis(3,3,4,4,5,5,6,6,6-nonafluorohexyl)- undergoes various chemical reactions, including:
Oxidation: This reaction can lead to the formation of silanols or siloxane bonds.
Reduction: Typically involves the reduction of siloxane bonds to silanes.
Substitution: Commonly occurs at the silicon atom, where alkyl or aryl groups can replace hydrogen atoms.
Common Reagents and Conditions
Oxidation: Reagents like hydrogen peroxide or ozone under controlled conditions.
Reduction: Reagents such as lithium aluminum hydride (LiAlH4) or sodium borohydride (NaBH4).
Substitution: Reagents like Grignard reagents or organolithium compounds.
Major Products
The major products formed from these reactions include various siloxane derivatives, which can be further functionalized for specific applications .
Scientific Research Applications
Surface Coatings
Trisiloxane compounds are widely used in surface coatings due to their excellent water-repellent properties. The nonafluorohexyl groups contribute to low surface energy and high hydrophobicity. This makes them ideal for:
- Waterproofing agents for textiles and building materials.
- Anti-fogging agents in automotive and optical applications.
These coatings enhance durability and performance in harsh environmental conditions.
Pharmaceutical Formulations
The unique siloxane structure provides biocompatibility and stability in pharmaceutical applications. Trisiloxane can be utilized as:
- Drug delivery systems , where it forms stable emulsions or suspensions.
- Excipient materials , aiding in the solubility and bioavailability of active pharmaceutical ingredients.
Personal Care Products
In the cosmetics industry, Trisiloxane is valued for its sensory properties:
- Skin conditioning agents that improve the feel and spreadability of creams and lotions.
- Hair care formulations , where it provides shine and reduces frizz without weighing hair down.
Specialty Chemicals
The compound is also a precursor for synthesizing various specialty chemicals:
- Used in the production of fluorosilicone polymers , which have applications in sealants and adhesives.
- Acts as a modifier in silicone rubber formulations to enhance thermal stability and mechanical properties.
Case Study 1: Water Repellent Coatings
A study conducted by researchers at a leading materials science institute demonstrated that coatings formulated with Trisiloxane exhibited superior water repellency compared to traditional silicone-based coatings. The treated surfaces showed a contact angle greater than 120 degrees after exposure to water for extended periods.
Case Study 2: Drug Delivery Systems
In a pharmaceutical research project published in the Journal of Controlled Release, Trisiloxane was incorporated into a nanoparticle formulation for targeted drug delivery. Results indicated enhanced drug solubility and controlled release profiles over time compared to conventional delivery methods.
Mechanism of Action
The mechanism of action of Trisiloxane, 1,1,3,5,5-pentamethyl-1,5-bis(3,3,4,4,5,5,6,6,6-nonafluorohexyl)- involves its interaction with various molecular targets and pathways:
Molecular Targets: The compound interacts with hydrophobic surfaces, reducing surface tension and enhancing wetting properties.
Pathways Involved: It modifies the surface energy of materials, leading to improved adhesion and spreading characteristics.
Comparison with Similar Compounds
Similar Compounds
1,1,1,3,5,5,5-Heptamethyltrisiloxane: Known for its use in agricultural adjuvants and cosmetic formulations.
1,3,5-Trivinyl-1,1,3,5,5-pentamethyltrisiloxane: Utilized in polymer synthesis and as a crosslinking agent.
1,1,1,5,5,5-Hexamethyl-3,3-bis(trimethylsiloxy)trisiloxane: Employed in the production of high-performance materials and coatings.
Uniqueness
Trisiloxane, 1,1,3,5,5-pentamethyl-1,5-bis(3,3,4,4,5,5,6,6,6-nonafluorohexyl)- stands out due to its unique combination of hydrophobic and oleophobic properties, making it highly effective in applications requiring extreme repellency to both water and oils .
Biological Activity
Trisiloxane, specifically 1,1,3,5,5-pentamethyl-1,5-bis(3,3,4,4,5,5,6,6,6-nonafluorohexyl)- , is a compound that has garnered attention in various fields due to its unique chemical structure and properties. This article explores its biological activity, drawing from diverse research findings and case studies.
Chemical Structure and Properties
The compound belongs to the class of trisiloxanes, characterized by a silicon-oxygen backbone with organic side groups. Its structure includes multiple fluorinated alkyl chains which enhance its hydrophobic properties and surface activity. The presence of these fluorinated groups makes it particularly interesting for applications in surfactants and emulsifiers.
Property | Value |
---|---|
Molecular Formula | C22H36F9O2Si3 |
Molecular Weight | 600.78 g/mol |
Solubility | Low in water |
Critical Micelle Concentration (CMC) | Variable with temperature |
Surface Activity
Trisiloxanes are known for their high surface activity compared to traditional hydrocarbon surfactants. The trimethylsiloxy groups contribute significantly to this property. Studies indicate that trisiloxanes can lower surface tension to values around 21 mN/m, making them effective in various applications such as detergents and coatings .
Toxicological Profile
Research on the toxicological effects of trisiloxanes indicates relatively low acute toxicity. For instance, studies involving analogs like D4 (cyclotetrasiloxane) suggest that while absorption through biological membranes is possible due to their low molecular weight, the overall systemic toxicity remains low .
Case Studies
- Environmental Impact : A study conducted on the environmental fate of trisiloxanes revealed that they can bioaccumulate in aquatic organisms but exhibit low toxicity at environmentally relevant concentrations. This finding is crucial for assessing their use in consumer products .
- Pharmaceutical Applications : Research has explored the use of trisiloxane surfactants in drug delivery systems. Their ability to form stable emulsions can enhance the bioavailability of hydrophobic drugs .
The biological mechanisms by which trisiloxanes exert their effects are primarily linked to their surfactant properties. They disrupt lipid bilayers in cell membranes, which can lead to altered permeability and potential cytotoxic effects at high concentrations. This property is leveraged in formulations designed for targeted delivery in pharmaceuticals.
Q & A
Basic Research Questions
Q. What are the recommended synthetic pathways and purification methods for trisiloxane derivatives with fluorinated alkyl chains?
Methodological Answer: The synthesis of fluorinated trisiloxanes typically involves hydrosilylation reactions between methylhydrosiloxane precursors and fluorinated alkenes, catalyzed by platinum-based catalysts (e.g., Karstedt catalyst). For example, the nonafluorohexyl group can be introduced via stoichiometric control to ensure regioselectivity. Post-synthesis purification often employs fractional distillation or preparative chromatography to isolate the target compound from unreacted siloxanes and byproducts. Critical parameters include inert atmosphere conditions (to prevent oxidation) and solvent selection (e.g., toluene or THF) to stabilize reactive intermediates .
Q. How can the structural integrity and purity of this trisiloxane compound be validated experimentally?
Methodological Answer: Combined analytical techniques are essential:
- NMR spectroscopy (¹H, ¹³C, ²⁹Si) confirms the siloxane backbone and fluorinated substituents. For instance, ²⁹Si NMR resolves Si–O–Si and Si–C linkages .
- FT-IR spectroscopy identifies Si–O–Si stretching (~1000–1100 cm⁻¹) and C–F vibrations (~1150–1250 cm⁻¹) .
- Mass spectrometry (ESI-TOF or MALDI-TOF) verifies molecular weight and isotopic patterns, critical for detecting trace impurities .
Q. What experimental protocols are used to assess the thermal stability of fluorinated trisiloxanes?
Methodological Answer:
- Thermogravimetric analysis (TGA) under nitrogen or air quantifies decomposition temperatures and residue formation. Fluorinated trisiloxanes typically exhibit higher thermal stability (>250°C) due to strong C–F bonds .
- Differential scanning calorimetry (DSC) identifies phase transitions (e.g., glass transitions or crystallization) influenced by fluorinated chain mobility .
Advanced Research Questions
Q. How does the fluorinated alkyl chain length (e.g., nonafluorohexyl) influence the aggregation behavior of trisiloxane surfactants in aqueous solutions?
Methodological Answer: Fluorinated chain length impacts critical micelle concentration (CMC) and aggregate morphology. Experimental approaches include:
- Surface tension measurements (using a Wilhelmy plate) to determine CMC. Shorter fluorinated chains (e.g., nonafluorohexyl) reduce CMC compared to non-fluorinated analogs .
- Small-angle X-ray scattering (SAXS) or dynamic light scattering (DLS) to characterize micelle size and shape. Longer fluorinated chains promote lamellar or vesicular structures, while shorter chains favor spherical micelles .
Q. What mechanisms explain the "superspreading" behavior of trisiloxane surfactants on hydrophobic surfaces?
Methodological Answer: Superspreading involves rapid Marangoni flows driven by surface tension gradients. Key experimental insights:
- High-speed microscopy tracks surfactant front propagation on hydrophobic substrates (e.g., PDMS). Trisiloxanes form metastable precursor films due to their low equilibrium surface tension (~20 mN/m) .
- Interfacial rheometry measures viscoelastic properties of surfactant-laden interfaces, correlating with spreading kinetics .
Q. How do fluorinated trisiloxanes interact with lipid bilayers, and what implications does this have for drug delivery systems?
Methodological Answer: Fluorinated trisiloxanes disrupt lipid packing via hydrophobic mismatch. Methodologies include:
- Fluorescence anisotropy using DPH probes to quantify membrane fluidity changes in model lipid bilayers (e.g., DPPC) .
- Cryo-TEM visualizes structural perturbations in liposomes exposed to trisiloxanes, revealing pore formation or bilayer fusion .
Q. Data Contradiction and Resolution
Q. How can researchers reconcile conflicting reports on the environmental persistence of fluorinated trisiloxanes?
Methodological Answer: Discrepancies arise from varying experimental conditions (e.g., pH, UV exposure). Standardized protocols are critical:
- Hydrolysis studies under controlled pH (e.g., pH 7.4 vs. 9.0) to quantify degradation products via LC-MS .
- Photolysis experiments using simulated sunlight (Xe arc lamps) to assess C–F bond stability and half-life .
Q. Why do some studies report trisiloxane surfactants forming stable monolayers while others observe rapid collapse?
Methodological Answer: Monolayer stability depends on fluorinated chain packing and subphase composition. Resolve contradictions via:
- Langmuir-Blodgett trough experiments with varying subphase ionic strength (e.g., NaCl or CaCl₂) to modulate surfactant headgroup interactions .
- Brewster angle microscopy (BAM) to image monolayer morphology in real-time, distinguishing between homogeneous films and collapse phases .
Q. Advanced Analytical Techniques
Q. What advanced spectroscopic methods are suitable for probing siloxane-fluorocarbon interactions in hybrid materials?
Methodological Answer:
- Solid-state NMR (¹⁹F and ²⁹Si) resolves spatial proximity between fluorinated chains and siloxane backbones in bulk materials .
- X-ray photoelectron spectroscopy (XPS) quantifies surface composition and bonding states (e.g., Si–O vs. Si–C) .
Q. How can computational modeling complement experimental studies of trisiloxane self-assembly?
Methodological Answer:
- Molecular dynamics (MD) simulations (e.g., GROMACS) model surfactant aggregation in explicit solvents, predicting CMC and aggregate size .
- Density functional theory (DFT) calculates electronic properties of fluorinated chains, guiding synthesis of analogs with tailored hydrophobicity .
Q. Safety and Handling
Q. What precautions are necessary when handling fluorinated trisiloxanes in laboratory settings?
Methodological Answer:
Properties
InChI |
InChI=1S/C17H23F18O2Si3/c1-38(36-39(2,3)8-6-10(18,19)12(22,23)14(26,27)16(30,31)32)37-40(4,5)9-7-11(20,21)13(24,25)15(28,29)17(33,34)35/h6-9H2,1-5H3 | |
---|---|---|
Source | PubChem | |
URL | https://pubchem.ncbi.nlm.nih.gov | |
Description | Data deposited in or computed by PubChem | |
InChI Key |
XVMMZZPJLIWHHM-UHFFFAOYSA-N | |
Source | PubChem | |
URL | https://pubchem.ncbi.nlm.nih.gov | |
Description | Data deposited in or computed by PubChem | |
Canonical SMILES |
C[Si](O[Si](C)(C)CCC(C(C(C(F)(F)F)(F)F)(F)F)(F)F)O[Si](C)(C)CCC(C(C(C(F)(F)F)(F)F)(F)F)(F)F | |
Source | PubChem | |
URL | https://pubchem.ncbi.nlm.nih.gov | |
Description | Data deposited in or computed by PubChem | |
Molecular Formula |
C17H23F18O2Si3 | |
Source | PubChem | |
URL | https://pubchem.ncbi.nlm.nih.gov | |
Description | Data deposited in or computed by PubChem | |
DSSTOX Substance ID |
DTXSID70895642 | |
Record name | 1,1,3,5,5-Pentamethyl-1,5-bis(3,3,4,4,5,5,6,6,6-nonafluorohexyl)trisiloxane | |
Source | EPA DSSTox | |
URL | https://comptox.epa.gov/dashboard/DTXSID70895642 | |
Description | DSSTox provides a high quality public chemistry resource for supporting improved predictive toxicology. | |
Molecular Weight |
685.6 g/mol | |
Source | PubChem | |
URL | https://pubchem.ncbi.nlm.nih.gov | |
Description | Data deposited in or computed by PubChem | |
CAS No. |
147986-73-0 | |
Record name | 1,1,3,5,5-Pentamethyl-1,5-bis(3,3,4,4,5,5,6,6,6-nonafluorohexyl)trisiloxane | |
Source | EPA DSSTox | |
URL | https://comptox.epa.gov/dashboard/DTXSID70895642 | |
Description | DSSTox provides a high quality public chemistry resource for supporting improved predictive toxicology. | |
Disclaimer and Information on In-Vitro Research Products
Please be aware that all articles and product information presented on BenchChem are intended solely for informational purposes. The products available for purchase on BenchChem are specifically designed for in-vitro studies, which are conducted outside of living organisms. In-vitro studies, derived from the Latin term "in glass," involve experiments performed in controlled laboratory settings using cells or tissues. It is important to note that these products are not categorized as medicines or drugs, and they have not received approval from the FDA for the prevention, treatment, or cure of any medical condition, ailment, or disease. We must emphasize that any form of bodily introduction of these products into humans or animals is strictly prohibited by law. It is essential to adhere to these guidelines to ensure compliance with legal and ethical standards in research and experimentation.