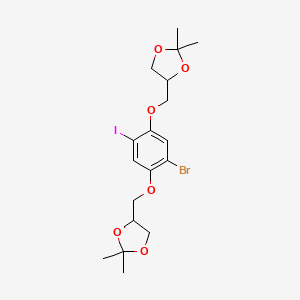
4,4'-(((2-Bromo-5-iodo-1,4-phenylene)bis(oxy))bis(methylene))bis(2,2-dimethyl-1,3-dioxolane)
- Click on QUICK INQUIRY to receive a quote from our team of experts.
- With the quality product at a COMPETITIVE price, you can focus more on your research.
Overview
Description
4,4’-(((2-Bromo-5-iodo-1,4-phenylene)bis(oxy))bis(methylene))bis(2,2-dimethyl-1,3-dioxolane) is a complex organic compound characterized by the presence of bromine and iodine atoms attached to a phenylene ring, which is further connected to dioxolane groups through oxy and methylene linkages
Preparation Methods
Synthetic Routes and Reaction Conditions
The synthesis of 4,4’-(((2-Bromo-5-iodo-1,4-phenylene)bis(oxy))bis(methylene))bis(2,2-dimethyl-1,3-dioxolane) typically involves the reaction of 5-bromo-1,3-difluoro-2-iodobenzene with 4-(tert-butyl)phenol in the presence of potassium carbonate as a base. The reaction is carried out in dry dimethylacetamide (DMA) under an argon atmosphere at 170°C for 36 hours . The resulting mixture is then cooled to room temperature and extracted with dichloromethane to obtain the desired product .
Industrial Production Methods
While specific industrial production methods for this compound are not well-documented, the general approach would involve scaling up the laboratory synthesis process. This would include optimizing reaction conditions, ensuring the availability of high-purity reagents, and implementing efficient purification techniques to achieve a high yield of the compound.
Chemical Reactions Analysis
Types of Reactions
4,4’-(((2-Bromo-5-iodo-1,4-phenylene)bis(oxy))bis(methylene))bis(2,2-dimethyl-1,3-dioxolane) can undergo various chemical reactions, including:
Substitution Reactions: The bromine and iodine atoms can be substituted with other functional groups using appropriate reagents.
Oxidation and Reduction Reactions: The phenylene ring and dioxolane groups can participate in oxidation and reduction reactions under suitable conditions.
Common Reagents and Conditions
Substitution Reactions: Reagents such as sodium iodide or potassium fluoride can be used to replace the halogen atoms.
Oxidation Reactions: Oxidizing agents like potassium permanganate or chromium trioxide can be employed.
Reduction Reactions: Reducing agents such as lithium aluminum hydride or sodium borohydride are commonly used.
Major Products Formed
The major products formed from these reactions depend on the specific reagents and conditions used. For example, substitution reactions may yield compounds with different functional groups replacing the bromine and iodine atoms, while oxidation and reduction reactions can modify the phenylene ring and dioxolane groups.
Scientific Research Applications
4,4’-(((2-Bromo-5-iodo-1,4-phenylene)bis(oxy))bis(methylene))bis(2,2-dimethyl-1,3-dioxolane) has several scientific research applications, including:
Chemistry: Used as a building block in the synthesis of more complex organic molecules.
Medicine: Investigated for its potential therapeutic properties and as a precursor for drug development.
Industry: Utilized in the production of advanced materials and as a reagent in various chemical processes.
Mechanism of Action
The mechanism of action of 4,4’-(((2-Bromo-5-iodo-1,4-phenylene)bis(oxy))bis(methylene))bis(2,2-dimethyl-1,3-dioxolane) involves its interaction with specific molecular targets and pathways. The presence of bromine and iodine atoms allows for unique reactivity, enabling the compound to participate in various chemical reactions. The dioxolane groups provide stability and enhance the compound’s solubility in organic solvents, facilitating its use in different applications.
Comparison with Similar Compounds
Similar Compounds
4,4’-((5-Bromo-2-iodo-1,3-phenylene)bis(oxy))bis(tert-butylbenzene): Similar structure but with tert-butylbenzene groups instead of dioxolane.
2,2’-Dimethyl-3,3’-bis[2-methyl-4-(2-acetamidoethylamino)-methyl-5-(3-cyanopyridin-5-yl-methoxy)phenoxymethyl]-1,1’-biphenyl: Contains similar phenylene and dioxolane groups but with different substituents.
Uniqueness
4,4’-(((2-Bromo-5-iodo-1,4-phenylene)bis(oxy))bis(methylene))bis(2,2-dimethyl-1,3-dioxolane) is unique due to the combination of bromine and iodine atoms on the phenylene ring and the presence of dioxolane groups. This unique structure imparts specific chemical properties and reactivity, making it valuable for various scientific and industrial applications.
Biological Activity
The compound 4,4'-(((2-Bromo-5-iodo-1,4-phenylene)bis(oxy))bis(methylene))bis(2,2-dimethyl-1,3-dioxolane) is a complex organic molecule that incorporates a dioxolane structure known for its diverse biological activities. This article reviews the biological activity of this compound based on available literature and research findings.
Chemical Structure and Properties
The compound features a central bis(phenylene) structure with ether linkages and dioxolane rings. The presence of bromine and iodine substituents on the phenylene moiety potentially enhances its biological activity by increasing lipophilicity and altering electronic properties.
Biological Activity Overview
Compounds containing dioxolane structures have been shown to exhibit a range of biological activities, including antibacterial, antifungal, antiviral, and anticancer properties. The specific biological activities of 4,4'-(((2-Bromo-5-iodo-1,4-phenylene)bis(oxy))bis(methylene))bis(2,2-dimethyl-1,3-dioxolane) are summarized in the following sections.
Antibacterial Activity
Research indicates that dioxolane derivatives can demonstrate significant antibacterial properties. For instance, a study on similar compounds showed effective inhibition against various Gram-positive and Gram-negative bacteria. The Minimum Inhibitory Concentration (MIC) values for some derivatives ranged from 625 to 1250 µg/mL against Staphylococcus aureus and Staphylococcus epidermidis .
Antifungal Activity
The antifungal activity of dioxolanes is also noteworthy. Compounds structurally related to the target molecule exhibited strong antifungal effects against Candida albicans, with several derivatives showing significant activity . This suggests that the target compound may possess similar antifungal capabilities.
Case Study 1: Synthesis and Evaluation of Dioxolanes
In a study examining the synthesis of new 1,3-dioxolanes, researchers found that compounds with ether or ester groups at specific positions displayed remarkable antibacterial and fungicidal activities. The synthesized compounds were tested against various microbial strains, demonstrating promising results that could be extrapolated to predict the activity of our target compound .
Compound | Activity Type | MIC (µg/mL) | Target Organism |
---|---|---|---|
Compound 1 | Antibacterial | 625 | S. aureus |
Compound 2 | Antifungal | - | C. albicans |
Compound 3 | Antibacterial | 1250 | S. epidermidis |
Case Study 2: Structure-Activity Relationship
A detailed examination of structure-activity relationships (SAR) among dioxolane derivatives revealed that modifications to the dioxolane ring significantly influenced biological efficacy. The presence of halogen substituents was correlated with enhanced antibacterial activity . This further supports the hypothesis that the halogenated structure of our target compound may confer similar advantages.
Crystallographic Studies
Crystallographic studies provide insights into the molecular geometry and potential interaction sites for biological targets. The crystal structure analysis of related compounds has revealed critical insights into their spatial configuration and bonding characteristics . Understanding these structural details is essential for predicting biological interactions.
Properties
Molecular Formula |
C18H24BrIO6 |
---|---|
Molecular Weight |
543.2 g/mol |
IUPAC Name |
4-[[2-bromo-4-[(2,2-dimethyl-1,3-dioxolan-4-yl)methoxy]-5-iodophenoxy]methyl]-2,2-dimethyl-1,3-dioxolane |
InChI |
InChI=1S/C18H24BrIO6/c1-17(2)23-9-11(25-17)7-21-15-6-14(20)16(5-13(15)19)22-8-12-10-24-18(3,4)26-12/h5-6,11-12H,7-10H2,1-4H3 |
InChI Key |
XRBMKIHGARNWMX-UHFFFAOYSA-N |
Canonical SMILES |
CC1(OCC(O1)COC2=CC(=C(C=C2I)OCC3COC(O3)(C)C)Br)C |
Origin of Product |
United States |
Disclaimer and Information on In-Vitro Research Products
Please be aware that all articles and product information presented on BenchChem are intended solely for informational purposes. The products available for purchase on BenchChem are specifically designed for in-vitro studies, which are conducted outside of living organisms. In-vitro studies, derived from the Latin term "in glass," involve experiments performed in controlled laboratory settings using cells or tissues. It is important to note that these products are not categorized as medicines or drugs, and they have not received approval from the FDA for the prevention, treatment, or cure of any medical condition, ailment, or disease. We must emphasize that any form of bodily introduction of these products into humans or animals is strictly prohibited by law. It is essential to adhere to these guidelines to ensure compliance with legal and ethical standards in research and experimentation.