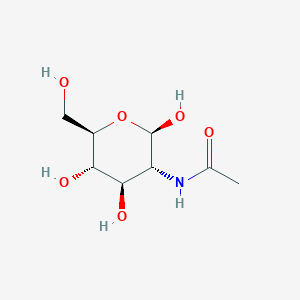
N-ACETYL-beta-D-GLUCOSAMINE
Overview
Description
2-Acetamido-2-deoxy-beta-D-glucopyranose, also known as N-acetylglucosamine, is a derivative of glucose. It is an amino sugar and a prominent component of various biopolymers, including chitin and glycosaminoglycans. This compound plays a crucial role in the biosynthesis of glycoproteins and glycolipids, which are essential for cellular communication and structural integrity.
Mechanism of Action
Target of Action
The primary target of 2-acetamido-2-deoxy-beta-D-glucopyranose is the Peptidoglycan recognition protein 3 in humans . This protein plays a crucial role in the immune response by recognizing peptidoglycans found in the cell walls of bacteria, leading to their elimination .
Mode of Action
It is known to interact with its target protein, potentially altering its function and leading to changes in the immune response .
Biochemical Pathways
Given its target, it is likely involved in the immune response pathways that deal with bacterial infections .
Result of Action
Given its target, it is likely to influence the immune response against bacterial infections .
Preparation Methods
Synthetic Routes and Reaction Conditions
The synthesis of N-ACETYL-beta-D-GLUCOSAMINE typically involves the acetylation of glucosamine. One common method is the reaction of glucosamine hydrochloride with acetic anhydride in the presence of a base such as sodium acetate. The reaction is carried out in an aqueous medium at a controlled temperature to yield the desired product .
Industrial Production Methods
Industrial production of this compound often employs enzymatic methods due to their efficiency and specificity. Enzymes such as chitinases and glucosaminidases are used to hydrolyze chitin, a natural polymer found in the exoskeletons of crustaceans and insects, to produce glucosamine. This glucosamine is then acetylated to form this compound .
Chemical Reactions Analysis
Types of Reactions
2-Acetamido-2-deoxy-beta-D-glucopyranose undergoes various chemical reactions, including:
Oxidation: It can be oxidized to form N-acetylglucosaminic acid.
Reduction: Reduction of the compound can yield N-acetylglucosamine alcohol.
Substitution: It can participate in substitution reactions where the acetyl group is replaced by other functional groups.
Common Reagents and Conditions
Oxidation: Common oxidizing agents include nitric acid and potassium permanganate.
Reduction: Sodium borohydride is often used as a reducing agent.
Substitution: Various nucleophiles can be used for substitution reactions, depending on the desired product.
Major Products
Oxidation: N-acetylglucosaminic acid
Reduction: N-acetylglucosamine alcohol
Substitution: Various substituted glucosamine derivatives
Scientific Research Applications
2-Acetamido-2-deoxy-beta-D-glucopyranose has a wide range of applications in scientific research:
Chemistry: It is used as a building block in the synthesis of complex carbohydrates and glycoproteins.
Biology: It plays a role in cell signaling and structural integrity of cells.
Medicine: It is used in the treatment of osteoarthritis and other joint disorders due to its role in cartilage formation.
Industry: It is used in the production of biodegradable plastics and as a stabilizer in various formulations.
Comparison with Similar Compounds
2-Acetamido-2-deoxy-beta-D-glucopyranose is unique due to its acetylated amino group, which distinguishes it from other similar compounds such as:
Glucosamine: Lacks the acetyl group and is less stable.
Galactosamine: Similar structure but differs in the orientation of the hydroxyl group at the C-4 position.
N-acetylgalactosamine: Similar to N-ACETYL-beta-D-GLUCOSAMINE but differs in the orientation of the hydroxyl group at the C-4 position.
Properties
IUPAC Name |
N-[(2R,3R,4R,5S,6R)-2,4,5-trihydroxy-6-(hydroxymethyl)oxan-3-yl]acetamide | |
---|---|---|
Source | PubChem | |
URL | https://pubchem.ncbi.nlm.nih.gov | |
Description | Data deposited in or computed by PubChem | |
InChI |
InChI=1S/C8H15NO6/c1-3(11)9-5-7(13)6(12)4(2-10)15-8(5)14/h4-8,10,12-14H,2H2,1H3,(H,9,11)/t4-,5-,6-,7-,8-/m1/s1 | |
Source | PubChem | |
URL | https://pubchem.ncbi.nlm.nih.gov | |
Description | Data deposited in or computed by PubChem | |
InChI Key |
OVRNDRQMDRJTHS-FMDGEEDCSA-N | |
Source | PubChem | |
URL | https://pubchem.ncbi.nlm.nih.gov | |
Description | Data deposited in or computed by PubChem | |
Canonical SMILES |
CC(=O)NC1C(C(C(OC1O)CO)O)O | |
Source | PubChem | |
URL | https://pubchem.ncbi.nlm.nih.gov | |
Description | Data deposited in or computed by PubChem | |
Isomeric SMILES |
CC(=O)N[C@@H]1[C@H]([C@@H]([C@H](O[C@H]1O)CO)O)O | |
Source | PubChem | |
URL | https://pubchem.ncbi.nlm.nih.gov | |
Description | Data deposited in or computed by PubChem | |
Molecular Formula |
C8H15NO6 | |
Source | PubChem | |
URL | https://pubchem.ncbi.nlm.nih.gov | |
Description | Data deposited in or computed by PubChem | |
DSSTOX Substance ID |
DTXSID301045979 | |
Record name | beta-N-Acetylglucosamine | |
Source | EPA DSSTox | |
URL | https://comptox.epa.gov/dashboard/DTXSID301045979 | |
Description | DSSTox provides a high quality public chemistry resource for supporting improved predictive toxicology. | |
Molecular Weight |
221.21 g/mol | |
Source | PubChem | |
URL | https://pubchem.ncbi.nlm.nih.gov | |
Description | Data deposited in or computed by PubChem | |
Physical Description |
Solid | |
Record name | beta-N-Acetylglucosamine | |
Source | Human Metabolome Database (HMDB) | |
URL | http://www.hmdb.ca/metabolites/HMDB0000803 | |
Description | The Human Metabolome Database (HMDB) is a freely available electronic database containing detailed information about small molecule metabolites found in the human body. | |
Explanation | HMDB is offered to the public as a freely available resource. Use and re-distribution of the data, in whole or in part, for commercial purposes requires explicit permission of the authors and explicit acknowledgment of the source material (HMDB) and the original publication (see the HMDB citing page). We ask that users who download significant portions of the database cite the HMDB paper in any resulting publications. | |
CAS No. |
14131-68-1, 72-87-7 | |
Record name | β-N-Acetylglucosamine | |
Source | CAS Common Chemistry | |
URL | https://commonchemistry.cas.org/detail?cas_rn=14131-68-1 | |
Description | CAS Common Chemistry is an open community resource for accessing chemical information. Nearly 500,000 chemical substances from CAS REGISTRY cover areas of community interest, including common and frequently regulated chemicals, and those relevant to high school and undergraduate chemistry classes. This chemical information, curated by our expert scientists, is provided in alignment with our mission as a division of the American Chemical Society. | |
Explanation | The data from CAS Common Chemistry is provided under a CC-BY-NC 4.0 license, unless otherwise stated. | |
Record name | D-Glucopyranose, 2-(acetylamino)-2-deoxy- | |
Source | ChemIDplus | |
URL | https://pubchem.ncbi.nlm.nih.gov/substance/?source=chemidplus&sourceid=0000072877 | |
Description | ChemIDplus is a free, web search system that provides access to the structure and nomenclature authority files used for the identification of chemical substances cited in National Library of Medicine (NLM) databases, including the TOXNET system. | |
Record name | N-Acetyl-beta-D-glucosamine | |
Source | ChemIDplus | |
URL | https://pubchem.ncbi.nlm.nih.gov/substance/?source=chemidplus&sourceid=0014131681 | |
Description | ChemIDplus is a free, web search system that provides access to the structure and nomenclature authority files used for the identification of chemical substances cited in National Library of Medicine (NLM) databases, including the TOXNET system. | |
Record name | beta-N-Acetylglucosamine | |
Source | EPA DSSTox | |
URL | https://comptox.epa.gov/dashboard/DTXSID301045979 | |
Description | DSSTox provides a high quality public chemistry resource for supporting improved predictive toxicology. | |
Record name | N-ACETYL-.BETA.-D-GLUCOSAMINE | |
Source | FDA Global Substance Registration System (GSRS) | |
URL | https://gsrs.ncats.nih.gov/ginas/app/beta/substances/8P59336F68 | |
Description | The FDA Global Substance Registration System (GSRS) enables the efficient and accurate exchange of information on what substances are in regulated products. Instead of relying on names, which vary across regulatory domains, countries, and regions, the GSRS knowledge base makes it possible for substances to be defined by standardized, scientific descriptions. | |
Explanation | Unless otherwise noted, the contents of the FDA website (www.fda.gov), both text and graphics, are not copyrighted. They are in the public domain and may be republished, reprinted and otherwise used freely by anyone without the need to obtain permission from FDA. Credit to the U.S. Food and Drug Administration as the source is appreciated but not required. | |
Record name | beta-N-Acetylglucosamine | |
Source | Human Metabolome Database (HMDB) | |
URL | http://www.hmdb.ca/metabolites/HMDB0000803 | |
Description | The Human Metabolome Database (HMDB) is a freely available electronic database containing detailed information about small molecule metabolites found in the human body. | |
Explanation | HMDB is offered to the public as a freely available resource. Use and re-distribution of the data, in whole or in part, for commercial purposes requires explicit permission of the authors and explicit acknowledgment of the source material (HMDB) and the original publication (see the HMDB citing page). We ask that users who download significant portions of the database cite the HMDB paper in any resulting publications. | |
Synthesis routes and methods
Procedure details
Retrosynthesis Analysis
AI-Powered Synthesis Planning: Our tool employs the Template_relevance Pistachio, Template_relevance Bkms_metabolic, Template_relevance Pistachio_ringbreaker, Template_relevance Reaxys, Template_relevance Reaxys_biocatalysis model, leveraging a vast database of chemical reactions to predict feasible synthetic routes.
One-Step Synthesis Focus: Specifically designed for one-step synthesis, it provides concise and direct routes for your target compounds, streamlining the synthesis process.
Accurate Predictions: Utilizing the extensive PISTACHIO, BKMS_METABOLIC, PISTACHIO_RINGBREAKER, REAXYS, REAXYS_BIOCATALYSIS database, our tool offers high-accuracy predictions, reflecting the latest in chemical research and data.
Strategy Settings
Precursor scoring | Relevance Heuristic |
---|---|
Min. plausibility | 0.01 |
Model | Template_relevance |
Template Set | Pistachio/Bkms_metabolic/Pistachio_ringbreaker/Reaxys/Reaxys_biocatalysis |
Top-N result to add to graph | 6 |
Feasible Synthetic Routes
Disclaimer and Information on In-Vitro Research Products
Please be aware that all articles and product information presented on BenchChem are intended solely for informational purposes. The products available for purchase on BenchChem are specifically designed for in-vitro studies, which are conducted outside of living organisms. In-vitro studies, derived from the Latin term "in glass," involve experiments performed in controlled laboratory settings using cells or tissues. It is important to note that these products are not categorized as medicines or drugs, and they have not received approval from the FDA for the prevention, treatment, or cure of any medical condition, ailment, or disease. We must emphasize that any form of bodily introduction of these products into humans or animals is strictly prohibited by law. It is essential to adhere to these guidelines to ensure compliance with legal and ethical standards in research and experimentation.