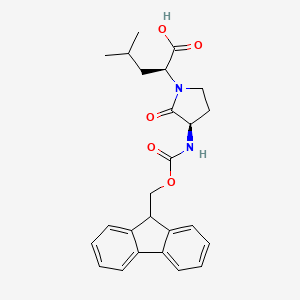
Fmoc-Freidinger's lactam
Overview
Description
Preparation Methods
Synthetic Routes and Reaction Conditions: Fmoc-Freidinger’s lactam can be synthesized through various methods, including solution-phase macrocyclization following solid-phase peptide synthesis (SPPS) of the linear precursor, SPPS and on-resin cyclization on the 2-chlorotrityl chloride (2-CTC) resin, and SPPS and on-resin cyclization by native chemical ligation on the amino-PEGA resin . These methods involve the formation of a lactam ring, which is a key step in the synthesis of many macrocyclic compounds.
Industrial Production Methods: Industrial production of Fmoc-Freidinger’s lactam typically involves large-scale SPPS techniques, which allow for the efficient and high-yield synthesis of the compound. The use of automated peptide synthesizers and advanced purification methods, such as high-performance liquid chromatography (HPLC), ensures the production of high-purity Fmoc-Freidinger’s lactam .
Chemical Reactions Analysis
Types of Reactions: Fmoc-Freidinger’s lactam undergoes various chemical reactions, including oxidation, reduction, and substitution. One notable reaction is the iodolactamization reaction, where alkenes react with iodine to generate halonium ions, followed by interaction with imine salts to obtain halogenated Fmoc-Freidinger’s lactam . Another reaction is the 1,3-dipolar cycloaddition (Kinugasa reaction) between alkynes and nitro compounds catalyzed by copper .
Common Reagents and Conditions: Common reagents used in the synthesis and reactions of Fmoc-Freidinger’s lactam include iodine, imine salts, alkynes, nitro compounds, and copper catalysts . The reactions are typically carried out under controlled conditions to ensure the desired product formation.
Major Products Formed: The major products formed from the reactions of Fmoc-Freidinger’s lactam include halogenated lactams and cycloaddition products, which can be further utilized in various applications, including peptide synthesis and drug development .
Scientific Research Applications
Drug Development
Fmoc-Freidinger's lactam has been instrumental in the creation of several therapeutic agents. Its applications include:
- Anticonvulsants : Compounds derived from γ-lactams have shown efficacy as anticonvulsants, with examples like Keppra™ and Briviact™, which are used in epilepsy treatment. These drugs leverage the structural properties of lactams to enhance potency and metabolic stability .
- Anticancer Agents : Modifications to the lactam core have led to the development of antitumor drugs that act as histone deacetylase inhibitors. The ability to modify substituents on the lactam ring allows for the fine-tuning of drug properties such as bioavailability and efficacy .
- Immunomodulatory Peptides : Research has demonstrated that incorporating this compound into peptide sequences can enhance their immunomodulatory effects, providing a pathway for developing novel therapeutic agents .
Peptide Synthesis
The synthesis of peptides utilizing this compound involves several innovative methodologies:
- Solid-Phase Peptide Synthesis : The lactam serves as a building block in solid-phase peptide synthesis protocols, allowing for the construction of complex peptide architectures with defined conformational constraints. This method enhances the efficiency and yield of peptide synthesis while maintaining structural integrity .
- Cyclization Techniques : The incorporation of this compound facilitates cyclization reactions that stabilize secondary structures within peptides. This is particularly important for peptides that require specific conformations to exhibit biological activity .
Structural Biology
In structural biology, this compound plays a crucial role in studying peptide conformation:
- Conformational Constraints : By introducing lactam bridges into peptide sequences, researchers can limit the degrees of freedom in peptide backbones, thus stabilizing bioactive conformations. This approach has been fundamental in understanding structure-activity relationships (SAR) within biologically active peptides .
- Peptide Mimics : The rigid structure provided by this compound allows for the design of peptidomimetics that can effectively mimic natural peptides while exhibiting enhanced stability and bioactivity. This has implications in drug design where mimicking natural processes is essential .
Case Studies
To illustrate these applications further, several case studies highlight the versatility and effectiveness of this compound:
Mechanism of Action
The mechanism of action of Fmoc-Freidinger’s lactam involves its ability to mimic the structure of natural peptides, allowing it to interact with specific molecular targets and pathways. The compound exerts its effects by binding to target proteins and enzymes, thereby modulating their activity and function . The conformational restriction provided by the lactam ring enhances the compound’s stability and bioactivity, making it an effective tool for studying biological processes and developing therapeutic agents .
Comparison with Similar Compounds
Similar Compounds: Similar compounds to Fmoc-Freidinger’s lactam include other lactams, such as β-lactams, γ-lactams, and δ-lactams. These compounds share a common lactam ring structure but differ in the size of the ring and the specific functional groups attached .
Uniqueness: Fmoc-Freidinger’s lactam is unique due to its conformational restriction and Fmoc protection, which provide enhanced stability and resistance to enzymatic degradation compared to other lactams . This makes it particularly valuable in peptide synthesis and drug development, where stability and bioactivity are critical factors .
Biological Activity
Introduction
Fmoc-Freidinger's lactam, a synthetic compound derived from the Freidinger lactam family, is characterized by its unique lactam structure featuring a 2-oxazolidinone ring. This structure contributes to its conformational rigidity and ability to mimic natural dipeptides, making it valuable in biochemical research and drug development. The compound's full chemical name is N-(9-fluorenylmethyloxycarbonyl)-2-azabicyclo[3.3.0]octan-3-one, with significant implications for enzyme interactions and cellular processes.
Chemical Structure and Properties
The molecular formula of this compound is C₁₄H₁₅NO₂, and its structure allows it to effectively mimic dipeptides while providing enhanced stability compared to other compounds. The conformationally restricted nature of the lactam enables it to interact with various biological targets, influencing enzyme activity such as that of proteases and peptidases.
Biological Activity
This compound exhibits a range of biological activities due to its ability to interact with enzymes and cellular receptors. Key areas of activity include:
- Enzyme Modulation : The compound has been shown to modulate the activity of various enzymes, particularly proteases and peptidases, which are crucial for protein metabolism.
- Cellular Function Influence : It influences several cellular functions, making it a valuable tool for studying biochemical pathways.
- Potential Therapeutic Applications : Due to its structural properties, this compound is explored for applications in drug discovery and development.
Interaction Studies
Research indicates that this compound interacts with various biomolecules, which is critical for understanding its therapeutic potential. Interaction studies have identified several key targets:
Biomolecule | Interaction Type | Biological Implication |
---|---|---|
Proteases | Competitive inhibition | Modulation of protein degradation pathways |
Peptidases | Substrate mimicry | Influence on peptide metabolism |
Receptors | Ligand-receptor interactions | Potential modulation of signaling pathways |
Case Studies
- Enzyme Inhibition : A study demonstrated that this compound acts as a competitive inhibitor for specific serine proteases, leading to altered substrate specificity and enzymatic activity.
- Cellular Assays : In vitro assays showed that this compound can modulate cell signaling pathways by interacting with G-protein coupled receptors (GPCRs), indicating potential applications in pharmacology.
- Peptide Synthesis Applications : The compound has been utilized in synthesizing conformationally constrained peptidomimetics, enhancing the stability and bioactivity of peptide-based drugs.
Synthesis Methods
The synthesis of this compound can be achieved through various methods, allowing flexibility in designing derivatives tailored for specific applications. Common synthetic pathways include:
- Reformatsky Reaction : Utilizes α-amino acid chlorides to generate the desired lactam structure.
- One-Pot Synthesis : Involves simultaneous protection and cyclization steps to produce the lactam efficiently.
Comparative Analysis with Related Compounds
This compound shares structural similarities with other compounds in the Freidinger lactam family. A comparative analysis highlights its unique features:
Compound Name | Structure Type | Unique Features |
---|---|---|
Freidinger Lactams | β-Lactams | Foundational structure for various analogs |
β-Amino Acids | Amino Acid Derivatives | Essential for peptide bond formation |
Prolyl-Leucyl-Glycine Amide | Peptidomimetic | Contains β-lactam moiety influencing β-turns |
Cyclic Dipeptides | Cyclic Peptides | Mimic natural peptides but with enhanced stability |
Q & A
Basic Research Questions
Q. What analytical techniques are recommended for confirming the structural integrity of Fmoc-Freidinger's lactam during synthesis?
- Methodological Answer : Employ nuclear magnetic resonance (NMR) spectroscopy (¹H and ¹³C) to verify the lactam ring and Fmoc group positioning. High-performance liquid chromatography (HPLC) with UV detection at 265–280 nm can assess purity, while mass spectrometry (MS) confirms molecular weight. For reproducibility, replicate analyses under standardized solvent conditions (e.g., deuterated DMF for NMR) .
Q. How does the lactam ring in this compound influence peptide backbone conformation?
- Methodological Answer : Use circular dichroism (CD) spectroscopy or X-ray crystallography to compare secondary structures (e.g., β-turns) in peptides synthesized with and without the lactam. Computational modeling (e.g., molecular dynamics simulations) can predict torsional strain and nonplanar amide resonance effects, as seen in β-lactam systems .
Q. What solvent systems optimize the solubility of this compound for solid-phase peptide synthesis (SPPS)?
- Methodological Answer : Test polar aprotic solvents (e.g., DMF, NMP) with 0.1% trifluoroacetic acid (TFA) to enhance solubility. Monitor coupling efficiency via Kaiser tests and adjust resin-swelling properties. Avoid aqueous buffers unless stabilized by co-solvents to prevent premature lactam hydrolysis .
Advanced Research Questions
Q. How can researchers resolve contradictions in reported enzymatic stability data for this compound?
- Methodological Answer : Replicate studies under controlled pH (5.0–7.4) and temperature (25–37°C) with standardized enzyme concentrations (e.g., β-lactamases, amidases). Use MALDI-TOF MS to identify degradation products (e.g., ring-opened species) and compare kinetics across enzyme classes. Apply mixed-effects statistical models to account for batch variability .
Q. What experimental designs are suitable for studying solvent polarity effects on lactam ring-opening kinetics?
- Methodological Answer : Conduct kinetic assays in solvents of varying polarity (e.g., DMSO, THF, water). Monitor reaction progress via HPLC or UV-Vis spectroscopy at λ = 220–240 nm (amide bond absorption). Use Arrhenius plots to calculate activation energies and solvent dielectric constants to correlate with rate constants .
Q. How can computational and experimental methods validate the role of torsional strain in this compound reactivity?
- Methodological Answer : Perform density functional theory (DFT) calculations to model torsional barriers and compare with X-ray crystallographic data. Experimentally, measure activation parameters (ΔH‡, ΔS‡) via variable-temperature NMR or stopped-flow kinetics. Cross-reference with β-lactam deformation studies .
Q. Data Analysis and Contradiction Management
Q. What statistical approaches are robust for analyzing lactam stability data with high variability?
- Methodological Answer : Use GraphPad Prism to apply nonlinear regression for kinetic curves and outlier detection (ROUT method). Report mean ± SEM with 95% confidence intervals. For conflicting datasets, perform meta-analysis using random-effects models to account for heterogeneity .
Q. How should researchers design controls to distinguish hydrolytic vs. enzymatic degradation pathways?
- Methodological Answer : Include negative controls with enzyme inhibitors (e.g., EDTA for metalloenzymes) and positive controls with known β-lactamase substrates. Use LC-MS/MS to quantify hydrolysis products (e.g., free Fmoc group) and compare degradation half-lives across conditions .
Q. Methodological Frameworks
Q. What criteria ensure a research question on lactam applications is both novel and feasible?
- Methodological Answer : Apply the FINER framework (Feasible, Interesting, Novel, Ethical, Relevant). For example, a study on lactam-modified antimicrobial peptides should address gaps in antibiotic resistance while using accessible techniques like SPPS and MIC assays .
Q. How can researchers optimize HPLC protocols for quantifying lactam degradation products?
- Methodological Answer : Use a C18 column with gradient elution (0.1% TFA in water/acetonitrile). Validate methods via spike-and-recovery experiments and calibration curves for lactam and hydrolyzed species. Include internal standards (e.g., deuterated analogs) to correct for matrix effects .
Properties
IUPAC Name |
(2S)-2-[(3R)-3-(9H-fluoren-9-ylmethoxycarbonylamino)-2-oxopyrrolidin-1-yl]-4-methylpentanoic acid | |
---|---|---|
Source | PubChem | |
URL | https://pubchem.ncbi.nlm.nih.gov | |
Description | Data deposited in or computed by PubChem | |
InChI |
InChI=1S/C25H28N2O5/c1-15(2)13-22(24(29)30)27-12-11-21(23(27)28)26-25(31)32-14-20-18-9-5-3-7-16(18)17-8-4-6-10-19(17)20/h3-10,15,20-22H,11-14H2,1-2H3,(H,26,31)(H,29,30)/t21-,22+/m1/s1 | |
Source | PubChem | |
URL | https://pubchem.ncbi.nlm.nih.gov | |
Description | Data deposited in or computed by PubChem | |
InChI Key |
MPIWMZZNSZSFPD-YADHBBJMSA-N | |
Source | PubChem | |
URL | https://pubchem.ncbi.nlm.nih.gov | |
Description | Data deposited in or computed by PubChem | |
Canonical SMILES |
CC(C)CC(C(=O)O)N1CCC(C1=O)NC(=O)OCC2C3=CC=CC=C3C4=CC=CC=C24 | |
Source | PubChem | |
URL | https://pubchem.ncbi.nlm.nih.gov | |
Description | Data deposited in or computed by PubChem | |
Isomeric SMILES |
CC(C)C[C@@H](C(=O)O)N1CC[C@H](C1=O)NC(=O)OCC2C3=CC=CC=C3C4=CC=CC=C24 | |
Source | PubChem | |
URL | https://pubchem.ncbi.nlm.nih.gov | |
Description | Data deposited in or computed by PubChem | |
Molecular Formula |
C25H28N2O5 | |
Source | PubChem | |
URL | https://pubchem.ncbi.nlm.nih.gov | |
Description | Data deposited in or computed by PubChem | |
Molecular Weight |
436.5 g/mol | |
Source | PubChem | |
URL | https://pubchem.ncbi.nlm.nih.gov | |
Description | Data deposited in or computed by PubChem | |
Retrosynthesis Analysis
AI-Powered Synthesis Planning: Our tool employs the Template_relevance Pistachio, Template_relevance Bkms_metabolic, Template_relevance Pistachio_ringbreaker, Template_relevance Reaxys, Template_relevance Reaxys_biocatalysis model, leveraging a vast database of chemical reactions to predict feasible synthetic routes.
One-Step Synthesis Focus: Specifically designed for one-step synthesis, it provides concise and direct routes for your target compounds, streamlining the synthesis process.
Accurate Predictions: Utilizing the extensive PISTACHIO, BKMS_METABOLIC, PISTACHIO_RINGBREAKER, REAXYS, REAXYS_BIOCATALYSIS database, our tool offers high-accuracy predictions, reflecting the latest in chemical research and data.
Strategy Settings
Precursor scoring | Relevance Heuristic |
---|---|
Min. plausibility | 0.01 |
Model | Template_relevance |
Template Set | Pistachio/Bkms_metabolic/Pistachio_ringbreaker/Reaxys/Reaxys_biocatalysis |
Top-N result to add to graph | 6 |
Feasible Synthetic Routes
Disclaimer and Information on In-Vitro Research Products
Please be aware that all articles and product information presented on BenchChem are intended solely for informational purposes. The products available for purchase on BenchChem are specifically designed for in-vitro studies, which are conducted outside of living organisms. In-vitro studies, derived from the Latin term "in glass," involve experiments performed in controlled laboratory settings using cells or tissues. It is important to note that these products are not categorized as medicines or drugs, and they have not received approval from the FDA for the prevention, treatment, or cure of any medical condition, ailment, or disease. We must emphasize that any form of bodily introduction of these products into humans or animals is strictly prohibited by law. It is essential to adhere to these guidelines to ensure compliance with legal and ethical standards in research and experimentation.