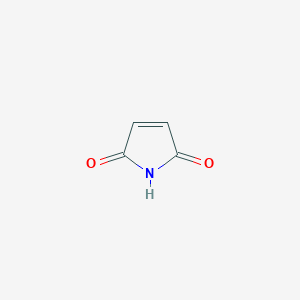
Maleimide
Overview
Description
Maleimide is a heterocyclic compound with the molecular formula C₄H₃NO₂, characterized by a five-membered ring containing two double bonds and a reactive imide group. Its structure enables diverse chemical modifications, making it a versatile scaffold in medicinal chemistry, polymer science, and materials engineering. This compound derivatives are widely utilized due to their electrophilic reactivity, particularly in Michael addition and Diels-Alder reactions, which facilitate covalent bonding with thiols or dienes . Applications range from anticancer agents and enzyme inhibitors to crosslinkers in polymer networks .
Preparation Methods
Synthetic Routes and Reaction Conditions: Maleimide and its derivatives are typically prepared from maleic anhydride by treatment with amines followed by dehydration . For example, the reaction of maleic anhydride with ammonia yields maleamic acid, which upon dehydration forms this compound. This process can be catalyzed by various dehydrating agents such as acetic anhydride or phosphorus pentoxide.
Industrial Production Methods: In industrial settings, this compound is produced through a similar process but on a larger scale. The reaction conditions are optimized to ensure high yield and purity. The use of continuous flow reactors and advanced purification techniques like crystallization and distillation are common in industrial production .
Chemical Reactions Analysis
Thiol-Maleimide Michael Addition: Primary Reaction Pathway
The maleimide-thiol reaction is a Michael addition forming a stable thioether bond (thiosuccinimide) (Figure 1). Key mechanistic insights include:
-
Driving forces : High reactivity arises from (a) ring strain in the this compound olefin (bond angle distortion) and (b) cis-conformation of carbonyl groups, enhancing electrophilicity .
-
pH dependence :
Solvent effects :
Solvent | Role in Reaction Kinetics | Source |
---|---|---|
Water/DMSO/DMF | Polar solvents promote thiolate formation, accelerating reaction without catalysts . | |
Chloroform | Slower kinetics due to reduced thiolate availability |
Mechanistic steps :
-
Deprotonation of thiol to thiolate (rate-limiting step in non-polar solvents).
-
Thiolate attack on this compound’s β-carbon.
Hydrolysis and Retro-Michael Reactions
This compound undergoes hydrolysis to non-reactive maleamic acid at pH >8.5 . The thiosuccinimide product is susceptible to:
-
Retro-Michael reactions : Thioether cleavage regenerates this compound, which reacts with other thiols (e.g., glutathione in vivo) .
-
Hydrolysis : Thiosuccinimide hydrolyzes to thiosuccinamic acid, reducing conjugate stability .
Kinetic parameters (substituted maleimides) :
Thiazine Formation with N-Terminal Cysteine
At pH ≥7.3 , maleimides react with N-terminal cysteine peptides to form six-membered thiazine rings via nucleophilic attack by the amine (Figure 2):
pH | Thiazine Conversion (24 h) |
---|---|
7.3 | 70% |
8.4 | 90% |
Data from . |
Optimal Conjugation Conditions
Parameter | Optimal Value | Conjugation Efficiency | Source |
---|---|---|---|
pH | 6.5–7.5 | >95% | |
This compound:Thiol Ratio | 2:1 (peptides), 5:1 (proteins) | 84–90% | |
Temperature | 25°C | Maximum rate |
Storage stability :
Inhibitors and Competing Agents
-
Thiol-containing agents : DTT, β-mercaptoethanol compete for this compound .
-
TCEP : Non-thiol reductant; compatible with this compound reactions .
Computational Insights into Selectivity
DFT studies reveal solvent and initiator effects on reaction pathways :
-
Base-initiated mechanism : Dominant in polar solvents (e.g., DMF) with amines as initiators.
-
Nucleophile-initiated pathway : Favored in non-polar solvents (e.g., chloroform) with phosphines.
-
Ion pair mechanism : Observed with strong bases (e.g., DABCO) in aprotic solvents.
Ternary reaction selectivity (N-methyl this compound + thiophenol + 1-hexanethiol):
Solvent | Initiator | Major Product |
---|---|---|
Chloroform | Et<sub>3</sub>N | Thiophenol adduct (95%) |
DMF | DABCO | 1-Hexanethiol adduct (82%) |
Data from . |
Emerging Strategies for Enhanced Stability
-
Substituted maleimides : Electron-withdrawing groups (e.g., -NO<sub>2</sub>) reduce retro-Michael rates .
-
This compound alternatives : Pyridazinediones show improved hydrolytic stability while retaining thiol selectivity .
-
Conjugation pH control : Buffers at pH 6.8–7.0 minimize amine competition and hydrolysis .
This synthesis of experimental and theoretical data underscores the balance between reaction efficiency and product stability in this compound chemistry. Recent advances in substituent design and pH control address historical limitations, expanding applications in targeted drug delivery and biomaterial engineering.
Scientific Research Applications
Drug Delivery Systems
1.1 Liposomal Drug Delivery
Maleimide-modified liposomes have emerged as an advanced platform for drug delivery. A study demonstrated that modifying liposomes with this compound-polyethylene glycol (PEG) significantly enhances their drug delivery efficiency compared to unmodified liposomes. The modified liposomes showed improved cellular uptake and a more potent antitumor effect in vivo when encapsulating doxorubicin. The biodistribution studies indicated that this compound-modified liposomes remained longer at the injection site, enhancing therapeutic efficacy without increasing cytotoxicity .
1.2 Antibiotic Delivery
Another innovative application involves this compound-conjugated PEGylated liposomal antibiotics designed to combat bacterial infections. This formulation not only enhances the intracellular uptake of antibiotics but also promotes wound healing by up-regulating genes related to cell cycle and angiogenesis. The this compound's ability to form covalent bonds with thiols allows for effective targeting of bacterial cell envelopes, thereby improving the therapeutic index of encapsulated antibiotics .
Antifungal Applications
Recent research has identified this compound compounds as potential antifungal agents against Candida albicans. A specific this compound derivative (MPD) demonstrated effective inhibition of virulence factors such as adhesion and biofilm formation with minimal cytotoxicity. This highlights this compound's role in developing antifungal therapies that reduce the risk of drug resistance and enhance treatment efficacy .
Bioconjugation Techniques
3.1 Click Chemistry
Maleimides are increasingly employed in bioconjugation through "click chemistry." Their ability to react with thiol groups makes them valuable for creating stable conjugates with proteins, peptides, and nucleic acids. For instance, this compound-modified oligonucleotides can be synthesized for targeted drug delivery, enhancing the specificity and effectiveness of therapeutic agents .
3.2 ELISA Development
In diagnostic applications, a this compound-based enzyme-linked immunosorbent assay (mELISA) has been developed. This method utilizes dithiol self-assembly on this compound plates to create stable monolayers for detecting various biological markers, showcasing this compound's utility in medical diagnostics .
Materials Science Applications
Maleimides are also significant in polymer science due to their diverse reactivity. They can be used to synthesize new polymers with tailored properties for applications in coatings, adhesives, and other materials requiring specific mechanical or chemical characteristics .
Summary Table of Applications
Mechanism of Action
The primary mechanism of action of maleimide involves its reactivity towards thiol groups in proteins and other biomolecules. The this compound group reacts with thiols to form stable thioether bonds, which is a key feature in bioconjugation . This reactivity is due to the electron-deficient nature of the this compound double bond, making it highly susceptible to nucleophilic attack by thiols .
Comparison with Similar Compounds
Comparison with Similar Compounds
Structural Analogues
Arylindolylmaleimides (e.g., PDA-66 vs. SB-216763)
- Structural Differences : PDA-66 features a 2-methylindole unit and a methylated maleimide group, whereas SB-216763 has a 2,4-dichloro substitution. The 4-acetyl group in PDA-66 enhances solubility and target affinity .
- Functional Activity : SB-216763 inhibits GSK3β with an IC₅₀ of 34.3 nM, while PDA-66 shows superior antiproliferative effects in acute lymphoblastoma cells due to optimized substituents .
Succinimide Derivatives (e.g., SPC-160001 vs. SPC-160002)
- Key Difference : Replacement of the this compound group in SPC-160002 with succinimide (SPC-160001) abolishes microtubule-stabilizing activity. This compound’s electrophilic α,β-unsaturated carbonyl enables covalent interactions with tubulin cysteine residues, critical for anticancer effects .
Compound | Structure | Microtubule Stabilization | IC₅₀ (Antiproliferative Activity) |
---|---|---|---|
SPC-160002 | This compound-containing | Yes | 0.8 µM (MCF-7 cells) |
SPC-160001 | Succinimide-containing | No | >10 µM |
3,4-Bis(arylthio)maleimides vs. 3-(Arylthio)maleimides
- Activity: Bis(arylthio) derivatives exhibit broader antimicrobial spectra against Gram-positive bacteria (MIC: 4–16 µg/mL) compared to monosubstituted analogs, likely due to enhanced lipophilicity and target engagement .
Functional Analogues in Enzyme Inhibition
This compound vs. Nikkomycin Z (Chitin Synthase Inhibitors)
- Potency : this compound-based compound H inhibits chitin synthase by 91%, comparable to nikkomycin Z (90%). The this compound fragment disrupts enzyme-substrate binding via hydrophobic interactions .
This compound vs. Alkyl Halides (Reactivity Comparison)
- Reactivity : Maleimides exhibit faster thiol-addition kinetics than primary alkyl halides (e.g., iodoacetamide) due to their conjugated electrophilic system. However, alkyl halides are less sterically hindered, enabling broader nucleophilic targeting .
This compound vs. Furan (Diels-Alder Crosslinking)
- Crosslinking Efficiency : this compound-furan systems achieve higher crosslinking density in chitosan hydrogels than furan-alone systems. For example, a this compound:furan molar ratio of 1:2 increases storage modulus (G’) by 150% compared to 1:1 ratios .
This compound-Modified Imidazoles vs. Conventional Imidazoles
- Curing Activity : this compound-modified imidazoles (e.g., IM-MA) eliminate NH groups, enhancing thermal stability in epoxy resins (Tg: 160°C vs. 120°C for unmodified imidazoles) .
Key Research Findings and Trends
Structure-Activity Relationships : Substitutions on the this compound ring (e.g., aryl, heterocyclic) significantly modulate biological potency. For example, 2-pyridyl substituents in N-(4-benzoylphenyl)maleimides enhance anticancer activity by 3-fold compared to unsubstituted analogs .
Reactivity Limitations : this compound’s susceptibility to hydrolysis and steric hindrance can reduce efficacy, prompting designs like PEGylated maleimides (e.g., DSPE-PEG 2000) for improved stability .
Therapeutic Potential: this compound derivatives like MIRA-1 reactivate mutant p53 in cancer cells, synergizing with chemotherapeutics (e.g., bortezomib) to overcome drug resistance .
Data Tables
Table 1. Enzyme Inhibitory Activities of this compound Derivatives
Compound | Target Enzyme | IC₅₀/Activity | Reference |
---|---|---|---|
SB-216763 | GSK3β | 34.3 nM | |
Compound H | Chitin Synthase | 91% inhibition | |
PDA-66 | GSK3β (Antiproliferative) | IC₅₀: 0.5 µM (CCRF-CEM cells) |
Table 2. Crosslinking Performance in Polymer Systems
System | This compound:Furan Ratio | Storage Modulus (G’) | Application |
---|---|---|---|
Chitosan-Hyaluronic Acid | 1:1 | 450 Pa | Tissue Engineering |
Chitosan-Hyaluronic Acid | 1:2 | 680 Pa | Tissue Engineering |
Biological Activity
Maleimide, a cyclic imide with a characteristic electrophilic nature, has garnered significant attention in the fields of medicinal chemistry and biochemistry due to its diverse biological activities. This article delves into the biological properties of this compound compounds, including their antibacterial, antifungal, anticancer activities, and their applications in drug delivery systems.
1. Chemical Structure and Properties
Maleimides are characterized by the general structure:
where and can be various alkyl or aryl groups. The electrophilic double bond in maleimides allows them to react specifically with thiol groups, making them valuable for conjugation in drug delivery systems and for targeting specific biomolecules.
2. Antibacterial Activity
Recent studies have highlighted the potential of this compound derivatives as antibacterial agents. For instance, a study on this compound-conjugated PEGylated liposomal antibiotics demonstrated enhanced efficacy against resistant bacterial strains such as E. coli and K. pneumoniae. The this compound groups facilitated covalent interactions with thiol-containing molecules on bacterial cell walls, thereby improving drug uptake and reducing minimum inhibitory concentrations (MIC) significantly compared to non-PEGylated formulations .
Table 1: Antibacterial Efficacy of this compound Compounds
3. Antifungal Activity
This compound compounds have also shown promising antifungal activity. A notable compound, 1-(4-methoxyphenyl)-1-hydro-pyrrole-2,5-dione (MPD), was found to inhibit virulence factors in Candida albicans, affecting processes such as adhesion and biofilm formation without significant cytotoxicity . In vivo studies using Galleria mellonella models indicated that treatment with MPD significantly prolonged survival during fungal infections.
Table 2: Antifungal Efficacy of this compound Compounds
Compound | Target Fungi | IC50 (µg/mL) | Effect on Virulence Factors |
---|---|---|---|
MPD | C. albicans | 0.5 | Inhibition of filamentation and biofilm |
BIM-IV | Sclerotinia sclerotiorum | 2 | Growth inhibition |
4. Anticancer Activity
The anticancer potential of maleimides has been extensively studied. Bisindolylmaleimides (BIMs), particularly BIM-IV, have been identified as potent inhibitors of protein kinase C (PKC), which is involved in various cancer signaling pathways . These compounds exhibit sub-micromolar inhibition against multiple PKC isoforms and have shown effectiveness against various cancer cell lines.
Table 3: Anticancer Efficacy of this compound Compounds
Compound | Cancer Cell Line | IC50 (µM) | Mechanism of Action |
---|---|---|---|
BIM-IV | MCF-7 | 0.1 | PKC inhibition |
Indolylthis compound B | HeLa | 0.5 | Inhibition of cell growth and migration |
The biological activities of maleimides can be attributed to several mechanisms:
- Thiol Interactions : The electrophilic nature allows maleimides to form covalent bonds with thiols on proteins, facilitating targeted drug delivery.
- Signal Transduction Modulation : Inhibitors like BIM-IV affect signaling pathways by inhibiting kinases involved in cell proliferation and survival.
- Biofilm Disruption : Compounds like MPD interfere with biofilm formation in fungi, reducing their pathogenicity.
Case Study 1: this compound-Conjugated Liposomes
A study investigated the use of this compound-conjugated PEGylated liposomes for antibiotic delivery. The results indicated a threefold increase in gene expression related to cell proliferation in treated cells compared to controls, showcasing enhanced therapeutic potential against resistant bacterial strains .
Case Study 2: Anti-Virulence Activity Against Candida albicans
Research on MPD demonstrated its ability to inhibit key virulence factors in C. albicans, leading to significant improvements in survival rates in infected models. The underlying mechanism involved modulation of intracellular signaling pathways affecting cAMP levels .
Q & A
Basic Research Questions
Q. What are the standard methods for quantifying maleimide groups in nanoparticle conjugates, and how do their sensitivities compare?
- Methodological Answer : this compound quantification typically employs spectrophotometry (absorbance at 302 nm, extinction coefficient: 620 M⁻¹ cm⁻¹) or fluorimetry. Fluorimetric assays, such as the Amplite® Kit, offer higher sensitivity (detection limit: 0.1 nM) by leveraging thiol-reactive probes . For nanoparticle systems, spectrophotometry may underestimate due to interference from protein absorbance, necessitating corrections using calibration curves or alternative methods like qPAINT (quantitative point accumulation for imaging in nanoscale topography) for ligand counting .
Q. How can researchers optimize this compound conjugation efficiency (CE%) in protein-polymer bioconjugates?
- Methodological Answer : CE% depends on polymer architecture and reaction conditions. For PEG-based systems, shorter spacer chains (e.g., PEG1k vs. PEG5k) reduce entanglement, improving ligand accessibility. Pre-functionalizing polymers with this compound via controlled radical polymerization (e.g., copper-mediated LRP) ensures >80% reactive chain ends. Validate CE% using spectrophotometry or thiol-specific assays (e.g., L-cysteine binding) and compare against theoretical values (Table S2†) .
Advanced Research Questions
Q. What experimental factors explain discrepancies between theoretical and observed this compound-ligand conjugation in PLGA–PEG nanoparticles?
- Methodological Answer : Only ~50% of this compound groups are surface-accessible due to PEG-PLGA miscibility, embedding some chains in the nanoparticle core. Quantify accessibility via qPAINT or competitive assays with small molecules (e.g., L-cysteine). For PEG5k systems, ligand availability drops to 6–9 ligands/NP vs. theoretical 77–293, highlighting the impact of PEG flexibility and molecular weight on steric hindrance .
Q. How can this compound-based bioconjugates address thiosuccinimide instability in therapeutic applications like antibody-drug conjugates (ADCs)?
- Methodological Answer : Thiosuccinimide linkages are prone to hydrolysis and retro-Michael reactions. Use next-generation maleimides (e.g., bromothis compound, DBCO-maleimide) for stabilized conjugates. For example, Diels-Alder "protected" maleimides enable reversible conjugation, while disulfide rebridging improves serum stability. Characterize stability via HPLC-SEC or mass spectrometry under physiological conditions (pH 7.4, 37°C) .
Q. What novel synthetic strategies improve this compound functionalization in hydrophilic polymers for biomedical applications?
- Methodological Answer : Copper-mediated living radical polymerization (LRP) allows precise control over this compound-terminated polymethacrylates (Mₙ = 4.1–35.4 kDa, PDI = 1.06–1.27). Two protocols achieve >80% this compound content: (1) Boc-protected amino initiators followed by deprotection, or (2) Diels-Alder adduct cleavage via retro-Diels-Alder. Validate functionality via conjugation with thiol-containing substrates (e.g., glutathione, BSA) in phosphate buffer (pH 6.8–7.4) .
Q. How can surface-enhanced Raman spectroscopy (SERS) be applied for semi-quantitative this compound detection in astrobiological samples?
- Methodological Answer : SERS detects this compound (2,5-pyrroledione) using colloidal silver nanoparticles as substrates. Prepare samples in a 60–120 µg/mL range, and acquire spectra under 785 nm excitation. Use principal component analysis (PCA) to differentiate this compound from interferents. Calibrate with a standard spectrum (reference provided in ), achieving a detection limit of ~10 µg/mL .
Q. Data Contradiction Analysis
Q. Why do spectrophotometric and fluorimetric assays yield conflicting this compound quantification results in complex matrices?
- Methodological Answer : Spectrophotometry underestimates this compound in protein-rich matrices due to overlapping absorbance (e.g., proteins at 280 nm). Fluorimetry, using this compound-specific probes (e.g., ThioGlo®), avoids this by targeting thiol reactivity. Cross-validate with orthogonal methods like HPLC or MALDI-MS, and normalize results to negative controls (e.g., non-maleimide polymers) .
Q. How should researchers reconcile low ligand availability (%) in this compound-functionalized nanoparticles despite high conjugation efficiency (%)?
- Methodological Answer : CE% measures total reacted this compound, while availability (%) reflects surface-accessible ligands. Use TEM-EDX or ToF-SIMS to map this compound distribution. For PEGylated systems, reduce spacer length (PEG1k > PEG5k) and this compound content (10% > 30%) to minimize PEG chain entanglement. Validate with qPAINT or SPR binding assays .
Q. Experimental Design Considerations
Q. What controls are essential when assessing this compound reactivity in biological buffers?
- Methodological Answer : Include (1) a no-thiol control to measure non-specific binding, (2) a this compound-free polymer control to assess background signal, and (3) a reducing agent (e.g., TCEP) to confirm thiol dependency. Perform kinetics studies (pH 6.8–7.4, 25–37°C) to optimize reaction time and minimize hydrolysis .
Q. How can researchers validate the specificity of this compound-thiol conjugation in vivo?
- Methodological Answer : Use dual-labeling strategies: (1) a fluorescent this compound probe (e.g., Alexa Fluor 647-maleimide) and (2) a competing non-reactive analog (e.g., succinimide). Image via confocal microscopy or quantify via flow cytometry. For in vivo studies, employ knockouts of target thiol proteins (e.g., serum albumin) to isolate conjugation specificity .
Properties
IUPAC Name |
pyrrole-2,5-dione | |
---|---|---|
Source | PubChem | |
URL | https://pubchem.ncbi.nlm.nih.gov | |
Description | Data deposited in or computed by PubChem | |
InChI |
InChI=1S/C4H3NO2/c6-3-1-2-4(7)5-3/h1-2H,(H,5,6,7) | |
Source | PubChem | |
URL | https://pubchem.ncbi.nlm.nih.gov | |
Description | Data deposited in or computed by PubChem | |
InChI Key |
PEEHTFAAVSWFBL-UHFFFAOYSA-N | |
Source | PubChem | |
URL | https://pubchem.ncbi.nlm.nih.gov | |
Description | Data deposited in or computed by PubChem | |
Canonical SMILES |
C1=CC(=O)NC1=O | |
Source | PubChem | |
URL | https://pubchem.ncbi.nlm.nih.gov | |
Description | Data deposited in or computed by PubChem | |
Molecular Formula |
C4H3NO2 | |
Source | PubChem | |
URL | https://pubchem.ncbi.nlm.nih.gov | |
Description | Data deposited in or computed by PubChem | |
Related CAS |
25721-74-8, Array | |
Record name | 1H-Pyrrole-2,5-dione, homopolymer | |
Source | CAS Common Chemistry | |
URL | https://commonchemistry.cas.org/detail?cas_rn=25721-74-8 | |
Description | CAS Common Chemistry is an open community resource for accessing chemical information. Nearly 500,000 chemical substances from CAS REGISTRY cover areas of community interest, including common and frequently regulated chemicals, and those relevant to high school and undergraduate chemistry classes. This chemical information, curated by our expert scientists, is provided in alignment with our mission as a division of the American Chemical Society. | |
Explanation | The data from CAS Common Chemistry is provided under a CC-BY-NC 4.0 license, unless otherwise stated. | |
Record name | Maleimide | |
Source | ChemIDplus | |
URL | https://pubchem.ncbi.nlm.nih.gov/substance/?source=chemidplus&sourceid=0000541593 | |
Description | ChemIDplus is a free, web search system that provides access to the structure and nomenclature authority files used for the identification of chemical substances cited in National Library of Medicine (NLM) databases, including the TOXNET system. | |
DSSTOX Substance ID |
DTXSID3049417 | |
Record name | Maleimide | |
Source | EPA DSSTox | |
URL | https://comptox.epa.gov/dashboard/DTXSID3049417 | |
Description | DSSTox provides a high quality public chemistry resource for supporting improved predictive toxicology. | |
Molecular Weight |
97.07 g/mol | |
Source | PubChem | |
URL | https://pubchem.ncbi.nlm.nih.gov | |
Description | Data deposited in or computed by PubChem | |
Physical Description |
White crystalline powder; [Alfa Aesar MSDS] | |
Record name | Maleimide | |
Source | Haz-Map, Information on Hazardous Chemicals and Occupational Diseases | |
URL | https://haz-map.com/Agents/20725 | |
Description | Haz-Map® is an occupational health database designed for health and safety professionals and for consumers seeking information about the adverse effects of workplace exposures to chemical and biological agents. | |
Explanation | Copyright (c) 2022 Haz-Map(R). All rights reserved. Unless otherwise indicated, all materials from Haz-Map are copyrighted by Haz-Map(R). No part of these materials, either text or image may be used for any purpose other than for personal use. Therefore, reproduction, modification, storage in a retrieval system or retransmission, in any form or by any means, electronic, mechanical or otherwise, for reasons other than personal use, is strictly prohibited without prior written permission. | |
Solubility |
9 [ug/mL] (The mean of the results at pH 7.4) | |
Record name | SID57264359 | |
Source | Burnham Center for Chemical Genomics | |
URL | https://pubchem.ncbi.nlm.nih.gov/bioassay/1996#section=Data-Table | |
Description | Aqueous solubility in buffer at pH 7.4 | |
Vapor Pressure |
0.00000277 [mmHg] | |
Record name | Maleimide | |
Source | Haz-Map, Information on Hazardous Chemicals and Occupational Diseases | |
URL | https://haz-map.com/Agents/20725 | |
Description | Haz-Map® is an occupational health database designed for health and safety professionals and for consumers seeking information about the adverse effects of workplace exposures to chemical and biological agents. | |
Explanation | Copyright (c) 2022 Haz-Map(R). All rights reserved. Unless otherwise indicated, all materials from Haz-Map are copyrighted by Haz-Map(R). No part of these materials, either text or image may be used for any purpose other than for personal use. Therefore, reproduction, modification, storage in a retrieval system or retransmission, in any form or by any means, electronic, mechanical or otherwise, for reasons other than personal use, is strictly prohibited without prior written permission. | |
CAS No. |
541-59-3 | |
Record name | Maleimide | |
Source | CAS Common Chemistry | |
URL | https://commonchemistry.cas.org/detail?cas_rn=541-59-3 | |
Description | CAS Common Chemistry is an open community resource for accessing chemical information. Nearly 500,000 chemical substances from CAS REGISTRY cover areas of community interest, including common and frequently regulated chemicals, and those relevant to high school and undergraduate chemistry classes. This chemical information, curated by our expert scientists, is provided in alignment with our mission as a division of the American Chemical Society. | |
Explanation | The data from CAS Common Chemistry is provided under a CC-BY-NC 4.0 license, unless otherwise stated. | |
Record name | Maleimide | |
Source | ChemIDplus | |
URL | https://pubchem.ncbi.nlm.nih.gov/substance/?source=chemidplus&sourceid=0000541593 | |
Description | ChemIDplus is a free, web search system that provides access to the structure and nomenclature authority files used for the identification of chemical substances cited in National Library of Medicine (NLM) databases, including the TOXNET system. | |
Record name | MALEIMIDE | |
Source | DTP/NCI | |
URL | https://dtp.cancer.gov/dtpstandard/servlet/dwindex?searchtype=NSC&outputformat=html&searchlist=13684 | |
Description | The NCI Development Therapeutics Program (DTP) provides services and resources to the academic and private-sector research communities worldwide to facilitate the discovery and development of new cancer therapeutic agents. | |
Explanation | Unless otherwise indicated, all text within NCI products is free of copyright and may be reused without our permission. Credit the National Cancer Institute as the source. | |
Record name | 1H-Pyrrole-2,5-dione | |
Source | EPA Chemicals under the TSCA | |
URL | https://www.epa.gov/chemicals-under-tsca | |
Description | EPA Chemicals under the Toxic Substances Control Act (TSCA) collection contains information on chemicals and their regulations under TSCA, including non-confidential content from the TSCA Chemical Substance Inventory and Chemical Data Reporting. | |
Record name | Maleimide | |
Source | EPA DSSTox | |
URL | https://comptox.epa.gov/dashboard/DTXSID3049417 | |
Description | DSSTox provides a high quality public chemistry resource for supporting improved predictive toxicology. | |
Record name | Maleimide | |
Source | European Chemicals Agency (ECHA) | |
URL | https://echa.europa.eu/substance-information/-/substanceinfo/100.007.990 | |
Description | The European Chemicals Agency (ECHA) is an agency of the European Union which is the driving force among regulatory authorities in implementing the EU's groundbreaking chemicals legislation for the benefit of human health and the environment as well as for innovation and competitiveness. | |
Explanation | Use of the information, documents and data from the ECHA website is subject to the terms and conditions of this Legal Notice, and subject to other binding limitations provided for under applicable law, the information, documents and data made available on the ECHA website may be reproduced, distributed and/or used, totally or in part, for non-commercial purposes provided that ECHA is acknowledged as the source: "Source: European Chemicals Agency, http://echa.europa.eu/". Such acknowledgement must be included in each copy of the material. ECHA permits and encourages organisations and individuals to create links to the ECHA website under the following cumulative conditions: Links can only be made to webpages that provide a link to the Legal Notice page. | |
Record name | MALEIMIDE | |
Source | FDA Global Substance Registration System (GSRS) | |
URL | https://gsrs.ncats.nih.gov/ginas/app/beta/substances/2519R1UGP8 | |
Description | The FDA Global Substance Registration System (GSRS) enables the efficient and accurate exchange of information on what substances are in regulated products. Instead of relying on names, which vary across regulatory domains, countries, and regions, the GSRS knowledge base makes it possible for substances to be defined by standardized, scientific descriptions. | |
Explanation | Unless otherwise noted, the contents of the FDA website (www.fda.gov), both text and graphics, are not copyrighted. They are in the public domain and may be republished, reprinted and otherwise used freely by anyone without the need to obtain permission from FDA. Credit to the U.S. Food and Drug Administration as the source is appreciated but not required. | |
Synthesis routes and methods I
Procedure details
Synthesis routes and methods II
Procedure details
Retrosynthesis Analysis
AI-Powered Synthesis Planning: Our tool employs the Template_relevance Pistachio, Template_relevance Bkms_metabolic, Template_relevance Pistachio_ringbreaker, Template_relevance Reaxys, Template_relevance Reaxys_biocatalysis model, leveraging a vast database of chemical reactions to predict feasible synthetic routes.
One-Step Synthesis Focus: Specifically designed for one-step synthesis, it provides concise and direct routes for your target compounds, streamlining the synthesis process.
Accurate Predictions: Utilizing the extensive PISTACHIO, BKMS_METABOLIC, PISTACHIO_RINGBREAKER, REAXYS, REAXYS_BIOCATALYSIS database, our tool offers high-accuracy predictions, reflecting the latest in chemical research and data.
Strategy Settings
Precursor scoring | Relevance Heuristic |
---|---|
Min. plausibility | 0.01 |
Model | Template_relevance |
Template Set | Pistachio/Bkms_metabolic/Pistachio_ringbreaker/Reaxys/Reaxys_biocatalysis |
Top-N result to add to graph | 6 |
Feasible Synthetic Routes
Disclaimer and Information on In-Vitro Research Products
Please be aware that all articles and product information presented on BenchChem are intended solely for informational purposes. The products available for purchase on BenchChem are specifically designed for in-vitro studies, which are conducted outside of living organisms. In-vitro studies, derived from the Latin term "in glass," involve experiments performed in controlled laboratory settings using cells or tissues. It is important to note that these products are not categorized as medicines or drugs, and they have not received approval from the FDA for the prevention, treatment, or cure of any medical condition, ailment, or disease. We must emphasize that any form of bodily introduction of these products into humans or animals is strictly prohibited by law. It is essential to adhere to these guidelines to ensure compliance with legal and ethical standards in research and experimentation.