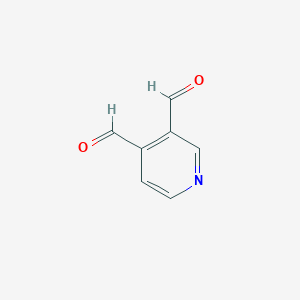
Pyridine-3,4-dicarbaldehyde
- Click on QUICK INQUIRY to receive a quote from our team of experts.
- With the quality product at a COMPETITIVE price, you can focus more on your research.
Overview
Description
Pyridine-3,4-dicarbaldehyde is an organic compound with the molecular formula C7H5NO2 It is a derivative of pyridine, where two hydrogen atoms on the pyridine ring are replaced by aldehyde groups at the 3 and 4 positions
Preparation Methods
Synthetic Routes and Reaction Conditions: Pyridine-3,4-dicarbaldehyde can be synthesized through several methods. One common approach involves the oxidation of pyridine derivatives. For instance, the oxidation of 3,4-dimethylpyridine using oxidizing agents such as potassium permanganate or chromium trioxide can yield this compound . Another method involves the Vilsmeier-Haack reaction, where pyridine is treated with a formylating agent like N,N-dimethylformamide (DMF) and phosphorus oxychloride (POCl3) to introduce the aldehyde groups .
Industrial Production Methods: Industrial production of this compound typically involves large-scale oxidation processes. The choice of oxidizing agent and reaction conditions can vary depending on the desired yield and purity of the product. Catalytic oxidation using metal catalysts such as palladium or platinum can also be employed to enhance the efficiency of the process .
Chemical Reactions Analysis
Types of Reactions: Pyridine-3,4-dicarbaldehyde undergoes various chemical reactions, including:
Common Reagents and Conditions:
Oxidation: Potassium permanganate, chromium trioxide, catalytic oxidation with palladium or platinum.
Reduction: Sodium borohydride, lithium aluminum hydride.
Substitution: Primary amines, secondary amines.
Major Products:
Oxidation: Pyridine-3,4-dicarboxylic acid.
Reduction: Pyridine-3,4-dimethanol.
Substitution: Imines, Schiff bases.
Scientific Research Applications
Pyridine-3,4-dicarbaldehyde has a wide range of applications in scientific research:
Mechanism of Action
The mechanism of action of pyridine-3,4-dicarbaldehyde and its derivatives often involves interactions with biological macromolecules. For instance, its antimicrobial activity is attributed to the formation of Schiff bases with microbial enzymes, disrupting their function . In anticancer applications, the compound can induce apoptosis in cancer cells by interacting with cellular proteins and DNA .
Comparison with Similar Compounds
Pyridine-3,4-dicarbaldehyde can be compared with other pyridinecarboxaldehydes, such as:
Pyridine-2-carboxaldehyde: Known for its use in coordination chemistry and as a precursor for various pharmaceuticals.
Pyridine-4-carboxaldehyde: Commonly used in the synthesis of heterocyclic compounds and as an intermediate in organic synthesis.
Pyridine-2,6-dicarbaldehyde: Utilized in the preparation of complex organic molecules and coordination compounds.
Uniqueness: this compound is unique due to its specific substitution pattern, which allows for distinct reactivity and applications compared to its isomers. Its ability to form stable Schiff bases and its potential in medicinal chemistry make it a valuable compound for research and industrial applications.
Properties
Molecular Formula |
C7H5NO2 |
---|---|
Molecular Weight |
135.12 g/mol |
IUPAC Name |
pyridine-3,4-dicarbaldehyde |
InChI |
InChI=1S/C7H5NO2/c9-4-6-1-2-8-3-7(6)5-10/h1-5H |
InChI Key |
PDZVRRLMPIFRHU-UHFFFAOYSA-N |
Canonical SMILES |
C1=CN=CC(=C1C=O)C=O |
Origin of Product |
United States |
Disclaimer and Information on In-Vitro Research Products
Please be aware that all articles and product information presented on BenchChem are intended solely for informational purposes. The products available for purchase on BenchChem are specifically designed for in-vitro studies, which are conducted outside of living organisms. In-vitro studies, derived from the Latin term "in glass," involve experiments performed in controlled laboratory settings using cells or tissues. It is important to note that these products are not categorized as medicines or drugs, and they have not received approval from the FDA for the prevention, treatment, or cure of any medical condition, ailment, or disease. We must emphasize that any form of bodily introduction of these products into humans or animals is strictly prohibited by law. It is essential to adhere to these guidelines to ensure compliance with legal and ethical standards in research and experimentation.