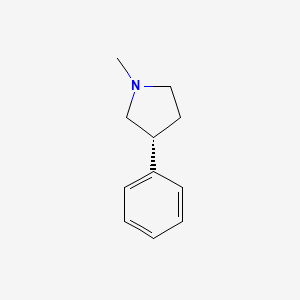
(R)-1-Methyl-3-phenylpyrrolidine
- Click on QUICK INQUIRY to receive a quote from our team of experts.
- With the quality product at a COMPETITIVE price, you can focus more on your research.
Overview
Description
®-1-Methyl-3-phenylpyrrolidine is a chiral compound that belongs to the class of pyrrolidines It is characterized by a pyrrolidine ring substituted with a methyl group at the first position and a phenyl group at the third position
Preparation Methods
Synthetic Routes and Reaction Conditions: The synthesis of ®-1-Methyl-3-phenylpyrrolidine typically involves the use of chiral catalysts or starting materials to ensure the desired enantiomer is obtained. One common method involves the asymmetric hydrogenation of 3-phenylpyrrolidine using a chiral rhodium catalyst. The reaction is carried out under hydrogen gas at elevated pressures and temperatures to achieve high enantioselectivity.
Industrial Production Methods: In an industrial setting, the production of ®-1-Methyl-3-phenylpyrrolidine may involve large-scale asymmetric synthesis using optimized catalysts and reaction conditions. Continuous flow reactors and other advanced technologies can be employed to enhance efficiency and yield.
Chemical Reactions Analysis
Types of Reactions: ®-1-Methyl-3-phenylpyrrolidine can undergo various chemical reactions, including:
Oxidation: The compound can be oxidized to form corresponding N-oxides or other oxidized derivatives.
Reduction: Reduction reactions can convert the compound into different reduced forms, such as amines.
Substitution: The phenyl group can participate in electrophilic aromatic substitution reactions, leading to various substituted derivatives.
Common Reagents and Conditions:
Oxidation: Common oxidizing agents include hydrogen peroxide and m-chloroperbenzoic acid.
Reduction: Reducing agents such as lithium aluminum hydride or sodium borohydride are often used.
Substitution: Electrophilic aromatic substitution reactions may involve reagents like bromine or nitric acid.
Major Products: The major products formed from these reactions depend on the specific conditions and reagents used. For example, oxidation may yield N-oxides, while substitution reactions can produce various phenyl-substituted derivatives.
Scientific Research Applications
®-1-Methyl-3-phenylpyrrolidine has a wide range of applications in scientific research:
Chemistry: It is used as a chiral building block in the synthesis of complex organic molecules.
Biology: The compound can serve as a ligand in the study of enzyme-substrate interactions and receptor binding.
Medicine: Research is ongoing to explore its potential as a pharmaceutical intermediate or active ingredient in drug development.
Industry: It is utilized in the production of fine chemicals and specialty materials.
Mechanism of Action
The mechanism of action of ®-1-Methyl-3-phenylpyrrolidine involves its interaction with specific molecular targets. The compound can bind to receptors or enzymes, modulating their activity and leading to various biological effects. The exact pathways and targets depend on the specific application and context of use.
Comparison with Similar Compounds
- (S)-1-Methyl-3-phenylpyrrolidine
- 1-Methyl-3-(4-methylphenyl)pyrrolidine
- 1-Methyl-3-(4-chlorophenyl)pyrrolidine
Comparison: ®-1-Methyl-3-phenylpyrrolidine is unique due to its specific chiral configuration, which can result in different biological activities and properties compared to its enantiomer (S)-1-Methyl-3-phenylpyrrolidine
Biological Activity
(R)-1-Methyl-3-phenylpyrrolidine is a chiral compound belonging to the pyrrolidine class, recognized for its unique biological activity, particularly in modulating neurotransmitter systems. This article delves into its synthesis, biological interactions, pharmacological profiles, and potential therapeutic applications.
Chemical Structure and Properties
This compound features a methyl group at the nitrogen atom and a phenyl group at the adjacent carbon atom. Its stereochemistry significantly influences its interaction with biological targets, enhancing its potential as a therapeutic agent. The compound's structure can be summarized as follows:
Property | Details |
---|---|
Molecular Formula | C₁₁H₁₅N |
Molecular Weight | 175.25 g/mol |
Chirality | (R) configuration |
Synthesis Methods
Various synthetic routes have been developed for this compound, emphasizing its accessibility in organic chemistry. Notable methods include:
- Chiral Pool Synthesis : Utilizing naturally occurring amino acids as starting materials.
- Asymmetric Synthesis : Employing catalysts to induce chirality during the formation of the pyrrolidine ring.
Biological Activity
Research indicates that this compound exhibits significant activity in modulating several neurotransmitter systems, particularly through interactions with receptors and enzymes.
Neurotransmitter Modulation
The compound has been shown to selectively engage with neurotransmitter receptors, influencing various central nervous system (CNS) functions. Key findings include:
- Dopamine Receptor Interaction : Studies suggest that this compound modulates dopamine levels, potentially impacting mood and cognitive functions.
- Sigma Receptor Activity : It has been identified as a positive allosteric modulator of sigma-1 receptors, which are implicated in neuroprotection and cognitive enhancement .
Pharmacological Profiles
The pharmacokinetic properties of this compound have been assessed in various preclinical models. These studies reveal:
Parameter | Value |
---|---|
Clearance (CL) | 41 ± 17 mL/min/kg (dog) |
Volume of Distribution (Vss) | 4.2 ± 3.3 L/kg (dog) |
Half-Life (t½) | 2.2 ± 0.8 h (dog) |
These metrics indicate a moderate clearance rate and volume of distribution, suggesting potential for effective systemic exposure .
Case Studies and Research Findings
- Anticoagulant Properties : Inhibitors derived from phenylpyrrolidine structures have shown promise as anticoagulants with minimal bleeding risks in preclinical models .
- Cognitive Enhancement : Comparative studies highlight that the (R) enantiomer exhibits superior antidepressant effects compared to its (S) counterpart, enhancing cognitive functions without significant side effects .
Comparative Analysis with Similar Compounds
To contextualize the biological activity of this compound, it is useful to compare it with structurally related compounds:
Compound Name | Structure Type | Unique Features |
---|---|---|
1-Methyl-3-pyridylpyrrolidine | Pyrrolidine | Different receptor selectivity |
(S)-1-Methyl-3-piperidinol | Piperidine | Higher potency but varied side effects |
1-Methyl-2-(4-methoxyphenyl)pyrrole | Pyrrole | Unique methoxy substitution affecting solubility |
This comparison illustrates the distinct pharmacological profiles that arise from minor structural variations within the pyrrolidine class.
Properties
Molecular Formula |
C11H15N |
---|---|
Molecular Weight |
161.24 g/mol |
IUPAC Name |
(3R)-1-methyl-3-phenylpyrrolidine |
InChI |
InChI=1S/C11H15N/c1-12-8-7-11(9-12)10-5-3-2-4-6-10/h2-6,11H,7-9H2,1H3/t11-/m0/s1 |
InChI Key |
YDNBZEZHOYJIKQ-NSHDSACASA-N |
Isomeric SMILES |
CN1CC[C@@H](C1)C2=CC=CC=C2 |
Canonical SMILES |
CN1CCC(C1)C2=CC=CC=C2 |
Origin of Product |
United States |
Disclaimer and Information on In-Vitro Research Products
Please be aware that all articles and product information presented on BenchChem are intended solely for informational purposes. The products available for purchase on BenchChem are specifically designed for in-vitro studies, which are conducted outside of living organisms. In-vitro studies, derived from the Latin term "in glass," involve experiments performed in controlled laboratory settings using cells or tissues. It is important to note that these products are not categorized as medicines or drugs, and they have not received approval from the FDA for the prevention, treatment, or cure of any medical condition, ailment, or disease. We must emphasize that any form of bodily introduction of these products into humans or animals is strictly prohibited by law. It is essential to adhere to these guidelines to ensure compliance with legal and ethical standards in research and experimentation.