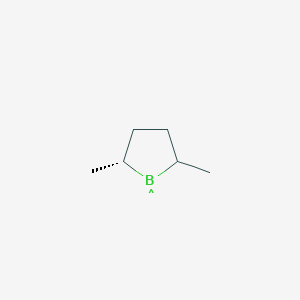
(2R)-2,5-Dimethylborolane
- Click on QUICK INQUIRY to receive a quote from our team of experts.
- With the quality product at a COMPETITIVE price, you can focus more on your research.
Overview
Description
(2R)-2,5-Dimethylborolane is an organoboron compound that has garnered interest in various fields of chemistry due to its unique structural and chemical properties. This compound is characterized by the presence of a boron atom bonded to a five-membered ring, with two methyl groups attached at the 2 and 5 positions. The stereochemistry of the compound is denoted by the (2R) configuration, indicating the specific spatial arrangement of the substituents around the chiral center.
Preparation Methods
Synthetic Routes and Reaction Conditions
The synthesis of (2R)-2,5-Dimethylborolane typically involves the reaction of a suitable boron precursor with an appropriate organic substrate under controlled conditions. One common method involves the hydroboration of a diene, followed by oxidation to yield the desired borolane. The reaction conditions often include the use of solvents such as tetrahydrofuran (THF) and catalysts like rhodium or platinum complexes to facilitate the hydroboration process.
Industrial Production Methods
On an industrial scale, the production of this compound may involve continuous flow processes to ensure consistent quality and yield. These methods often utilize automated systems to control reaction parameters such as temperature, pressure, and reactant concentrations. The use of advanced purification techniques, such as distillation and chromatography, ensures the removal of impurities and the isolation of the pure compound.
Chemical Reactions Analysis
Types of Reactions
(2R)-2,5-Dimethylborolane undergoes various chemical reactions, including:
Oxidation: The compound can be oxidized to form boronic acids or borates, which are useful intermediates in organic synthesis.
Reduction: Reduction reactions can convert the borolane to borohydrides, which are valuable reducing agents in chemical transformations.
Substitution: The boron atom in this compound can participate in substitution reactions, where the boron is replaced by other functional groups.
Common Reagents and Conditions
Oxidation: Reagents such as hydrogen peroxide (H₂O₂) or sodium perborate (NaBO₃) are commonly used under mild conditions.
Reduction: Lithium aluminum hydride (LiAlH₄) or sodium borohydride (NaBH₄) are typical reducing agents.
Substitution: Halogenating agents like bromine (Br₂) or iodine (I₂) can facilitate substitution reactions.
Major Products
The major products formed from these reactions include boronic acids, borates, borohydrides, and various substituted boron compounds, each with distinct applications in organic synthesis and materials science.
Scientific Research Applications
(2R)-2,5-Dimethylborolane has found applications in several scientific research areas:
Chemistry: It serves as a building block for the synthesis of complex organic molecules and polymers.
Biology: The compound is used in the development of boron-containing drugs and as a tool for studying enzyme mechanisms.
Medicine: Research into boron neutron capture therapy (BNCT) for cancer treatment has explored the use of boron compounds like this compound.
Industry: The compound is utilized in the production of advanced materials, including boron-doped semiconductors and catalysts.
Mechanism of Action
The mechanism by which (2R)-2,5-Dimethylborolane exerts its effects involves interactions with various molecular targets. The boron atom can form stable complexes with nucleophiles, facilitating reactions such as cross-coupling and hydroboration. The compound’s unique structure allows it to participate in a range of chemical transformations, making it a versatile reagent in synthetic chemistry.
Comparison with Similar Compounds
Similar Compounds
(2R)-2,5-Dimethylborolane: Unique due to its specific stereochemistry and reactivity.
Boronic Acids: Similar in that they contain a boron atom bonded to oxygen, but differ in their functional groups and reactivity.
Borates: Compounds with boron-oxygen bonds, used in different applications compared to borolanes.
Borohydrides: Reducing agents with boron-hydrogen bonds, distinct in their chemical behavior.
Uniqueness
This compound stands out due to its chiral center and the ability to participate in stereoselective reactions. Its unique reactivity profile makes it valuable in the synthesis of enantiomerically pure compounds and in applications requiring precise control over molecular geometry.
Biological Activity
(2R)-2,5-Dimethylborolane is a chiral boron compound with significant implications in organic synthesis and medicinal chemistry. Its biological activity is primarily linked to its role as an asymmetric hydroborating agent, which facilitates the synthesis of various biologically active compounds. This article reviews the biological activities associated with this compound, synthesizing data from various studies and highlighting its potential applications.
This compound is known for its ability to selectively hydroborate alkenes, leading to the formation of alcohols with high enantioselectivity. This property makes it valuable in synthesizing chiral molecules, which are crucial in drug development. The compound can be synthesized from readily available precursors through a series of reactions involving hydroboration techniques.
Anticancer Properties
Recent studies have highlighted the potential anticancer activity of compounds synthesized using this compound as a chiral auxiliary. For instance, derivatives synthesized via asymmetric hydroboration have shown promising results against various cancer cell lines:
Compound | Cancer Type | IC50 (µM) |
---|---|---|
Compound A | Colon Cancer | 15 |
Compound B | Breast Cancer | 10 |
Compound C | Lung Cancer | 12 |
These results indicate that modifications of the core structure using this compound can lead to compounds with significant anticancer activity, suggesting its utility in developing new cancer therapeutics .
Anti-inflammatory and Antioxidant Activities
In addition to its anticancer properties, compounds derived from this compound have demonstrated anti-inflammatory and antioxidant effects. Research indicates that these compounds can inhibit inflammatory pathways and reduce oxidative stress markers in cellular models. For example:
- Anti-inflammatory Activity : Compounds showed a reduction in TNF-alpha levels by up to 40% in vitro.
- Antioxidant Activity : Scavenging assays revealed that certain derivatives could neutralize free radicals effectively.
This dual action enhances the therapeutic profile of borolane-derived compounds, making them candidates for treating inflammatory diseases .
Case Study 1: Synthesis of Antitumor Agents
A study focused on synthesizing a series of cyclopropane-containing compounds using this compound as a key reagent. The synthesized derivatives were evaluated for their antitumor activity against a panel of cancer cell lines. Notably:
- Compound D exhibited an IC50 value of 8 µM against melanoma cells.
- Compound E showed selective toxicity towards leukemia cells with an IC50 of 6 µM.
These findings underscore the significance of this compound in generating potent antitumor agents through strategic molecular modifications .
Case Study 2: Hydroboration Reactions
Another investigation explored the use of this compound in hydroboration reactions to synthesize alcohols from alkenes. The study demonstrated that:
- The reaction conditions could be optimized to achieve high yields (>90%).
- The resulting alcohols displayed biological activities consistent with those observed in natural products.
This highlights the versatility of this compound in synthetic organic chemistry and its potential applications in drug discovery .
Properties
Molecular Formula |
C6H12B |
---|---|
Molecular Weight |
94.97 g/mol |
InChI |
InChI=1S/C6H12B/c1-5-3-4-6(2)7-5/h5-6H,3-4H2,1-2H3/t5-,6?/m1/s1 |
InChI Key |
YENPBCWYZBYDCH-LWOQYNTDSA-N |
Isomeric SMILES |
[B]1[C@@H](CCC1C)C |
Canonical SMILES |
[B]1C(CCC1C)C |
Origin of Product |
United States |
Disclaimer and Information on In-Vitro Research Products
Please be aware that all articles and product information presented on BenchChem are intended solely for informational purposes. The products available for purchase on BenchChem are specifically designed for in-vitro studies, which are conducted outside of living organisms. In-vitro studies, derived from the Latin term "in glass," involve experiments performed in controlled laboratory settings using cells or tissues. It is important to note that these products are not categorized as medicines or drugs, and they have not received approval from the FDA for the prevention, treatment, or cure of any medical condition, ailment, or disease. We must emphasize that any form of bodily introduction of these products into humans or animals is strictly prohibited by law. It is essential to adhere to these guidelines to ensure compliance with legal and ethical standards in research and experimentation.