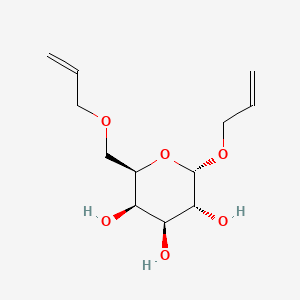
(2S,3R,4S,5R,6R)-2-prop-2-enoxy-6-(prop-2-enoxymethyl)oxane-3,4,5-triol
- Click on QUICK INQUIRY to receive a quote from our team of experts.
- With the quality product at a COMPETITIVE price, you can focus more on your research.
Overview
Description
(2S,3R,4S,5R,6R)-2-prop-2-enoxy-6-(prop-2-enoxymethyl)oxane-3,4,5-triol is a complex organic compound belonging to the class of oxanes. This compound is characterized by its multiple hydroxyl groups and prop-2-enoxy substituents, making it a versatile molecule in various chemical reactions and applications. Its unique stereochemistry, indicated by the specific configuration of its chiral centers, plays a crucial role in its reactivity and interaction with other molecules.
Preparation Methods
Synthetic Routes and Reaction Conditions
The synthesis of (2S,3R,4S,5R,6R)-2-prop-2-enoxy-6-(prop-2-enoxymethyl)oxane-3,4,5-triol typically involves the protection of hydroxyl groups, selective functionalization, and subsequent deprotection steps. One common synthetic route includes the following steps:
Protection of Hydroxyl Groups: The hydroxyl groups of a suitable precursor, such as a hexose sugar, are protected using protecting groups like acetals or silyl ethers.
Introduction of Prop-2-enoxy Groups: The protected intermediate is then subjected to nucleophilic substitution reactions with prop-2-enol derivatives under basic conditions to introduce the prop-2-enoxy groups.
Deprotection: The protecting groups are removed under acidic or basic conditions to yield the final product.
Industrial Production Methods
Industrial production of this compound may involve similar synthetic routes but on a larger scale. The process optimization focuses on maximizing yield and purity while minimizing production costs. Techniques such as continuous flow reactors and automated synthesis platforms are often employed to enhance efficiency and scalability.
Chemical Reactions Analysis
Types of Reactions
(2S,3R,4S,5R,6R)-2-prop-2-enoxy-6-(prop-2-enoxymethyl)oxane-3,4,5-triol undergoes various chemical reactions, including:
Oxidation: The hydroxyl groups can be oxidized to form carbonyl compounds using oxidizing agents like pyridinium chlorochromate or Dess-Martin periodinane.
Reduction: The compound can be reduced to form alkanes or alcohols using reducing agents such as lithium aluminum hydride or sodium borohydride.
Substitution: The prop-2-enoxy groups can be substituted with other nucleophiles under appropriate conditions.
Common Reagents and Conditions
Oxidation: Pyridinium chlorochromate, Dess-Martin periodinane, and other mild oxidizing agents.
Reduction: Lithium aluminum hydride, sodium borohydride, and catalytic hydrogenation.
Substitution: Nucleophiles such as halides, thiols, and amines under basic or acidic conditions.
Major Products Formed
Oxidation: Formation of aldehydes, ketones, or carboxylic acids.
Reduction: Formation of alkanes or alcohols.
Substitution: Formation of substituted oxanes with various functional groups.
Scientific Research Applications
(2S,3R,4S,5R,6R)-2-prop-2-enoxy-6-(prop-2-enoxymethyl)oxane-3,4,5-triol has diverse applications in scientific research, including:
Chemistry: Used as a building block for the synthesis of more complex molecules and as a chiral auxiliary in asymmetric synthesis.
Biology: Studied for its potential role in biological processes and as a probe for investigating enzyme-substrate interactions.
Medicine: Explored for its potential therapeutic properties, including anti-inflammatory and anticancer activities.
Industry: Utilized in the production of specialty chemicals, polymers, and materials with unique properties.
Mechanism of Action
The mechanism of action of (2S,3R,4S,5R,6R)-2-prop-2-enoxy-6-(prop-2-enoxymethyl)oxane-3,4,5-triol involves its interaction with specific molecular targets and pathways. The compound’s hydroxyl groups and prop-2-enoxy substituents enable it to form hydrogen bonds and other interactions with enzymes, receptors, and other biomolecules. These interactions can modulate the activity of enzymes, alter signal transduction pathways, and influence cellular processes.
Comparison with Similar Compounds
Similar Compounds
(2S,3R,4S,5R,6R)-2-methoxy-6-(methoxymethyl)oxane-3,4,5-triol: Similar structure but with methoxy groups instead of prop-2-enoxy groups.
(2S,3R,4S,5R,6R)-2-ethoxy-6-(ethoxymethyl)oxane-3,4,5-triol: Similar structure but with ethoxy groups instead of prop-2-enoxy groups.
Uniqueness
(2S,3R,4S,5R,6R)-2-prop-2-enoxy-6-(prop-2-enoxymethyl)oxane-3,4,5-triol is unique due to its specific stereochemistry and the presence of prop-2-enoxy groups. These features confer distinct reactivity and interaction profiles compared to similar compounds with different substituents. The prop-2-enoxy groups provide additional sites for chemical modification and functionalization, enhancing the compound’s versatility in various applications.
Biological Activity
The compound (2S,3R,4S,5R,6R)-2-prop-2-enoxy-6-(prop-2-enoxymethyl)oxane-3,4,5-triol is a complex organic molecule with significant potential in various biological applications. Its unique structural features may confer a range of biological activities, including antioxidant, anticoagulant, and antimicrobial properties. This article examines the biological activity of this compound based on existing research findings and case studies.
Structural Characteristics
The molecular formula of the compound is C12H20O6, with a molecular weight of 260.28 g/mol. Its stereochemistry and functional groups are pivotal in determining its biological interactions.
1. Antioxidant Properties
Compounds similar in structure to this compound often exhibit antioxidant activity . This activity is primarily due to their ability to scavenge free radicals and reduce oxidative stress in biological systems.
Research Findings:
- In vitro studies have demonstrated that compounds with similar functional groups can effectively neutralize reactive oxygen species (ROS), which are implicated in various diseases including cancer and neurodegenerative disorders.
2. Anticoagulant Activity
The compound may also possess anticoagulant properties , which can be crucial in managing conditions related to blood clotting.
Case Studies:
- Related compounds have shown efficacy in modulating blood coagulation pathways by inhibiting specific enzymes involved in the coagulation cascade. This suggests that this compound could similarly influence these pathways.
3. Antimicrobial Activity
The presence of multiple functional groups within the compound could enhance its antimicrobial activity by facilitating interactions with microbial membranes or enzymes.
Experimental Evidence:
- Preliminary studies indicated that structurally similar compounds exhibited significant antimicrobial effects against a range of pathogens. The ability to disrupt microbial cell membranes is a potential mechanism for this activity.
Interaction Studies
Understanding how this compound interacts with biological targets is crucial for optimizing its efficacy and safety profiles.
Docking Simulations:
Recent docking studies have suggested that this compound binds effectively to various protein targets involved in metabolic pathways and disease processes. For example:
Target Protein | Binding Affinity (kcal/mol) |
---|---|
RAD50 | -7.8 |
These simulations indicate a strong potential for therapeutic applications in conditions where these proteins are dysregulated .
Comparative Analysis
To contextualize the biological activity of this compound within the broader field of similar compounds:
Compound Name | Structural Features | Unique Properties |
---|---|---|
D-glucose | Simple hexose | Primary energy source |
D-fructose | Ketose | Sweet flavor; energy source |
D-mannose | Epimer of glucose | Immune support properties |
This comparison highlights how the complex structure of this compound may provide enhanced bioactivity compared to simpler sugars.
Properties
Molecular Formula |
C12H20O6 |
---|---|
Molecular Weight |
260.28 g/mol |
IUPAC Name |
(2S,3R,4S,5R,6R)-2-prop-2-enoxy-6-(prop-2-enoxymethyl)oxane-3,4,5-triol |
InChI |
InChI=1S/C12H20O6/c1-3-5-16-7-8-9(13)10(14)11(15)12(18-8)17-6-4-2/h3-4,8-15H,1-2,5-7H2/t8-,9+,10+,11-,12+/m1/s1 |
InChI Key |
OMKXRHOKCCXNSL-IIRVCBMXSA-N |
Isomeric SMILES |
C=CCOC[C@@H]1[C@@H]([C@@H]([C@H]([C@H](O1)OCC=C)O)O)O |
Canonical SMILES |
C=CCOCC1C(C(C(C(O1)OCC=C)O)O)O |
Origin of Product |
United States |
Disclaimer and Information on In-Vitro Research Products
Please be aware that all articles and product information presented on BenchChem are intended solely for informational purposes. The products available for purchase on BenchChem are specifically designed for in-vitro studies, which are conducted outside of living organisms. In-vitro studies, derived from the Latin term "in glass," involve experiments performed in controlled laboratory settings using cells or tissues. It is important to note that these products are not categorized as medicines or drugs, and they have not received approval from the FDA for the prevention, treatment, or cure of any medical condition, ailment, or disease. We must emphasize that any form of bodily introduction of these products into humans or animals is strictly prohibited by law. It is essential to adhere to these guidelines to ensure compliance with legal and ethical standards in research and experimentation.