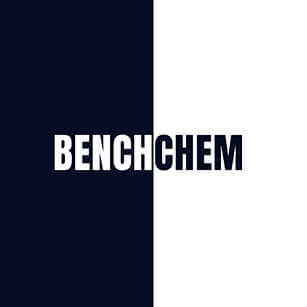
Europium Oxide
- Click on QUICK INQUIRY to receive a quote from our team of experts.
- With the quality product at a COMPETITIVE price, you can focus more on your research.
Overview
Description
Europium oxide is a chemical compound composed of europium and oxygen. It is commonly found in two oxidation states: europium(II) oxide and europium(III) oxide. Europium(III) oxide, with the chemical formula Eu₂O₃, is the most stable form and appears as a white to light-pink solid. This compound is widely used in various applications, including as a red or blue phosphor in television sets and fluorescent lamps, and as an activator for yttrium-based phosphors .
Synthetic Routes and Reaction Conditions:
Reduction of Europium(III) Oxide: Europium(II) oxide can be synthesized by reducing europium(III) oxide with metallic europium at high temperatures.
Carbothermic Reduction: Another method involves the carbothermic reduction of europium(III) oxide using carbon at temperatures ranging from 1300°C to 1500°C.
Industrial Production Methods:
Thermal Decomposition: Europium(III) oxide can be produced industrially by the thermal decomposition of europium nitrate.
Types of Reactions:
Oxidation: Europium(II) oxide can be oxidized to europium(III) oxide in the presence of oxygen.
Reduction: Europium(III) oxide can be reduced to europium(II) oxide using reducing agents such as hydrogen or carbon.
Substitution: this compound can react with acids to form europium salts, such as europium chloride.
Common Reagents and Conditions:
Oxidation: Oxygen or air at elevated temperatures.
Reduction: Hydrogen gas or carbon at high temperatures.
Substitution: Acids like hydrochloric acid under controlled conditions.
Major Products:
Oxidation: Europium(III) oxide (Eu₂O₃).
Reduction: Europium(II) oxide (EuO).
Substitution: Europium salts (e.g., europium chloride).
Scientific Research Applications
Europium oxide has a wide range of scientific research applications:
Chemistry: Used as a phosphor in various lighting and display technologies due to its luminescent properties.
Biology and Medicine: this compound is used in bioimaging and as a contrast agent in medical imaging due to its fluorescent properties.
Radiation Shielding: this compound is used in the development of radiation-shielding materials due to its high density and ability to absorb gamma rays.
Mechanism of Action
The mechanism by which europium oxide exerts its effects is primarily through its luminescent properties. When europium ions are excited by ultraviolet light, they emit visible light, making them useful in various lighting and display applications. The molecular targets and pathways involved include the excitation of europium ions to higher energy levels, followed by the emission of light as the ions return to their ground state .
Comparison with Similar Compounds
- Samarium(III) oxide (Sm₂O₃)
- Gadolinium(III) oxide (Gd₂O₃)
- Ytterbium(III) oxide (Yb₂O₃)
Comparison:
- Luminescent Properties: Europium oxide is unique in its strong red and blue luminescence, making it particularly valuable in display technologies. In contrast, samarium and gadolinium oxides have different luminescent properties and are used in other specialized applications .
- Oxidation States: this compound can exist in both +2 and +3 oxidation states, whereas some similar compounds, like gadolinium oxide, primarily exist in the +3 state .
- Applications: While this compound is widely used in lighting and display technologies, similar compounds like samarium oxide are used in nuclear reactors and gadolinium oxide in magnetic resonance imaging (MRI) contrast agents .
This compound stands out due to its unique luminescent properties and versatility in various scientific and industrial applications.
Properties
CAS No. |
12770-85-3 |
---|---|
Molecular Formula |
Eu2O |
Molecular Weight |
0 |
Origin of Product |
United States |
Disclaimer and Information on In-Vitro Research Products
Please be aware that all articles and product information presented on BenchChem are intended solely for informational purposes. The products available for purchase on BenchChem are specifically designed for in-vitro studies, which are conducted outside of living organisms. In-vitro studies, derived from the Latin term "in glass," involve experiments performed in controlled laboratory settings using cells or tissues. It is important to note that these products are not categorized as medicines or drugs, and they have not received approval from the FDA for the prevention, treatment, or cure of any medical condition, ailment, or disease. We must emphasize that any form of bodily introduction of these products into humans or animals is strictly prohibited by law. It is essential to adhere to these guidelines to ensure compliance with legal and ethical standards in research and experimentation.