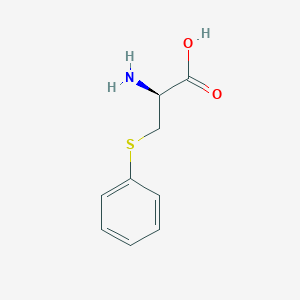
S-phenyl-D-cysteine
- Click on QUICK INQUIRY to receive a quote from our team of experts.
- With the quality product at a COMPETITIVE price, you can focus more on your research.
Overview
Description
(S)-2-Amino-3-(phenylthio)propanoic acid is an organic compound characterized by the presence of an amino group, a phenylthio group, and a carboxylic acid group This compound is a derivative of cysteine, where the sulfur atom is bonded to a phenyl group
Preparation Methods
Synthetic Routes and Reaction Conditions: The synthesis of (S)-2-Amino-3-(phenylthio)propanoic acid typically involves the following steps:
Starting Material: The synthesis begins with L-cysteine, a naturally occurring amino acid.
Thioether Formation: L-cysteine undergoes a reaction with a phenylthiol in the presence of a suitable catalyst to form the thioether linkage. Common catalysts include transition metal complexes.
Purification: The product is then purified using techniques such as recrystallization or chromatography to obtain the desired (S)-2-Amino-3-(phenylthio)propanoic acid.
Industrial Production Methods: In an industrial setting, the production of (S)-2-Amino-3-(phenylthio)propanoic acid may involve large-scale batch reactors where the reaction conditions are optimized for yield and purity. Continuous flow reactors may also be employed to enhance efficiency and scalability.
Chemical Reactions Analysis
Types of Reactions: (S)-2-Amino-3-(phenylthio)propanoic acid can undergo various chemical reactions, including:
Oxidation: The sulfur atom can be oxidized to form sulfoxides or sulfones using oxidizing agents such as hydrogen peroxide or m-chloroperbenzoic acid.
Reduction: The compound can be reduced to form thiols using reducing agents like lithium aluminum hydride.
Substitution: The amino group can participate in substitution reactions, forming derivatives with different functional groups.
Common Reagents and Conditions:
Oxidation: Hydrogen peroxide, m-chloroperbenzoic acid.
Reduction: Lithium aluminum hydride, sodium borohydride.
Substitution: Various alkylating or acylating agents under basic or acidic conditions.
Major Products:
Oxidation: Sulfoxides, sulfones.
Reduction: Thiols.
Substitution: N-substituted derivatives.
Scientific Research Applications
(S)-2-Amino-3-(phenylthio)propanoic acid has a wide range of applications in scientific research:
Chemistry: It is used as a building block for the synthesis of more complex molecules and as a ligand in coordination chemistry.
Biology: The compound is studied for its role in enzymatic reactions and as a potential inhibitor of certain enzymes.
Medicine: Research is ongoing into its potential therapeutic applications, including its use as a precursor for drug development.
Industry: It is used in the synthesis of specialty chemicals and as an intermediate in the production of pharmaceuticals.
Mechanism of Action
The mechanism of action of (S)-2-Amino-3-(phenylthio)propanoic acid involves its interaction with specific molecular targets:
Enzymatic Inhibition: The compound can inhibit certain enzymes by binding to their active sites, thereby blocking substrate access.
Pathways Involved: It may affect metabolic pathways involving sulfur-containing amino acids and their derivatives.
Comparison with Similar Compounds
Cysteine: A naturally occurring amino acid with a thiol group instead of a phenylthio group.
Methionine: Another sulfur-containing amino acid with a methylthio group.
S-Phenylcysteine: A compound similar to (S)-2-Amino-3-(phenylthio)propanoic acid but with different stereochemistry.
Uniqueness: (S)-2-Amino-3-(phenylthio)propanoic acid is unique due to the presence of the phenylthio group, which imparts distinct chemical and biological properties compared to other sulfur-containing amino acids. This uniqueness makes it a valuable compound for research and industrial applications.
Properties
Molecular Formula |
C9H11NO2S |
---|---|
Molecular Weight |
197.26 g/mol |
IUPAC Name |
(2S)-2-amino-3-phenylsulfanylpropanoic acid |
InChI |
InChI=1S/C9H11NO2S/c10-8(9(11)12)6-13-7-4-2-1-3-5-7/h1-5,8H,6,10H2,(H,11,12)/t8-/m1/s1 |
InChI Key |
XYUBQWNJDIAEES-MRVPVSSYSA-N |
Isomeric SMILES |
C1=CC=C(C=C1)SC[C@H](C(=O)O)N |
Canonical SMILES |
C1=CC=C(C=C1)SCC(C(=O)O)N |
Origin of Product |
United States |
Disclaimer and Information on In-Vitro Research Products
Please be aware that all articles and product information presented on BenchChem are intended solely for informational purposes. The products available for purchase on BenchChem are specifically designed for in-vitro studies, which are conducted outside of living organisms. In-vitro studies, derived from the Latin term "in glass," involve experiments performed in controlled laboratory settings using cells or tissues. It is important to note that these products are not categorized as medicines or drugs, and they have not received approval from the FDA for the prevention, treatment, or cure of any medical condition, ailment, or disease. We must emphasize that any form of bodily introduction of these products into humans or animals is strictly prohibited by law. It is essential to adhere to these guidelines to ensure compliance with legal and ethical standards in research and experimentation.