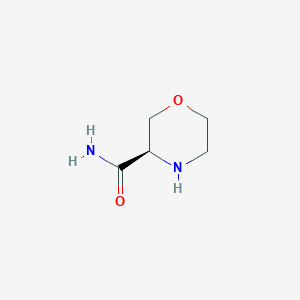
(3R)-Morpholine-3-carboxamide
- Click on QUICK INQUIRY to receive a quote from our team of experts.
- With the quality product at a COMPETITIVE price, you can focus more on your research.
Overview
Description
(3R)-Morpholine-3-carboxamide is a chiral compound with significant applications in various fields, including chemistry, biology, and medicine. This compound is characterized by its morpholine ring, which is a six-membered ring containing both nitrogen and oxygen atoms, and a carboxamide group attached to the third carbon atom in the ring. The (3R) designation indicates the specific stereochemistry of the molecule, which can influence its reactivity and interactions with biological targets.
Preparation Methods
Synthetic Routes and Reaction Conditions
The synthesis of (3R)-Morpholine-3-carboxamide typically involves the formation of the morpholine ring followed by the introduction of the carboxamide group. One common method involves the cyclization of an appropriate amino alcohol precursor under acidic or basic conditions to form the morpholine ring. Subsequent acylation of the resulting morpholine with a suitable carboxylic acid derivative, such as an acid chloride or anhydride, yields the desired carboxamide.
Industrial Production Methods
Industrial production of this compound may involve optimized synthetic routes to ensure high yield and purity. This often includes the use of catalytic processes and continuous flow reactors to enhance reaction efficiency and scalability. Additionally, purification techniques such as crystallization, distillation, and chromatography are employed to isolate the final product.
Chemical Reactions Analysis
Types of Reactions
(3R)-Morpholine-3-carboxamide can undergo various chemical reactions, including:
Oxidation: The compound can be oxidized using oxidizing agents such as hydrogen peroxide or potassium permanganate, leading to the formation of oxidized derivatives.
Reduction: Reduction reactions can be carried out using reducing agents like lithium aluminum hydride or sodium borohydride to produce reduced forms of the compound.
Common Reagents and Conditions
Oxidation: Hydrogen peroxide, potassium permanganate, and other oxidizing agents under acidic or basic conditions.
Reduction: Lithium aluminum hydride, sodium borohydride, and other reducing agents in solvents like ether or tetrahydrofuran.
Substitution: Nucleophiles such as alkyl halides, acyl halides, and other electrophiles in the presence of a base or acid catalyst.
Major Products Formed
The major products formed from these reactions depend on the specific reagents and conditions used. For example, oxidation may yield hydroxylated or ketone derivatives, while reduction can produce amine or alcohol derivatives. Substitution reactions can introduce various functional groups, leading to a wide range of substituted morpholine derivatives.
Scientific Research Applications
(3R)-Morpholine-3-carboxamide has numerous applications in scientific research, including:
Chemistry: Used as a building block for the synthesis of more complex molecules, including pharmaceuticals and agrochemicals.
Biology: Serves as a ligand in the study of enzyme-substrate interactions and protein-ligand binding studies.
Medicine: Investigated for its potential therapeutic effects, including its role as an intermediate in the synthesis of drugs targeting specific biological pathways.
Industry: Utilized in the production of specialty chemicals and materials with unique properties.
Mechanism of Action
The mechanism of action of (3R)-Morpholine-3-carboxamide involves its interaction with specific molecular targets, such as enzymes or receptors. The compound’s stereochemistry plays a crucial role in its binding affinity and specificity. For example, the (3R) configuration may allow for optimal interactions with active sites of enzymes, leading to inhibition or activation of enzymatic activity. The pathways involved can include signal transduction, metabolic regulation, and other cellular processes.
Comparison with Similar Compounds
Similar Compounds
(3S)-Morpholine-3-carboxamide: The enantiomer of (3R)-Morpholine-3-carboxamide, differing only in the stereochemistry at the third carbon atom.
Morpholine-4-carboxamide: A structural isomer with the carboxamide group attached to the fourth carbon atom instead of the third.
N-Methylmorpholine: A derivative with a methyl group attached to the nitrogen atom in the morpholine ring.
Uniqueness
This compound is unique due to its specific stereochemistry, which can significantly influence its biological activity and chemical reactivity. This makes it a valuable compound for studying stereochemical effects in various applications, including drug design and development.
Biological Activity
(3R)-Morpholine-3-carboxamide is a compound that has garnered attention due to its diverse biological activities, particularly in pharmacology. This article aims to provide a comprehensive overview of its biological activity, including its mechanisms of action, therapeutic potential, and relevant case studies.
Chemical Structure and Properties
This compound belongs to the morpholine family, characterized by a six-membered ring containing one nitrogen atom. The specific configuration at the 3-position (R) is crucial for its biological activity. The compound can be represented as follows:
The biological activity of this compound is primarily attributed to its role as an antagonist of prokineticin receptors. Prokineticins are involved in various physiological processes, including pain modulation, neurogenesis, and circadian rhythms. Disruption in prokineticin signaling has been linked to neurological and psychiatric disorders, making this compound a candidate for therapeutic intervention .
Table 1: Summary of Biological Activities
Case Studies
- Prokineticin Receptor Antagonism : In a study examining the effects of morpholine derivatives on prokineticin receptors, this compound showed significant antagonistic activity. This was evidenced by its ability to block receptor-mediated signaling pathways in vitro, which could translate into therapeutic benefits for conditions like anxiety and depression .
- Anti-inflammatory Effects : Another investigation focused on the compound's anti-inflammatory properties. It was found that this compound reduced the secretion of pro-inflammatory cytokines in human cell lines, suggesting potential applications in treating inflammatory diseases .
- Neuroprotection : Research highlighted the neuroprotective capabilities of this compound against oxidative stress-induced damage in neuronal cultures. This suggests a role in neurodegenerative disease management, although further studies are necessary to elucidate the underlying mechanisms .
Structure-Activity Relationship (SAR)
Understanding the structure-activity relationship is vital for optimizing the efficacy and safety profile of this compound. Modifications at various positions on the morpholine ring can significantly affect its biological activity.
Table 2: Structure-Activity Relationship Insights
Modification Location | Change Made | Effect on Activity |
---|---|---|
2-position | Addition of alkyl group | Increased receptor selectivity |
4-position | Hydroxyl substitution | Enhanced anti-inflammatory effects |
5-position | Aromatic ring incorporation | Improved neuroprotective properties |
Properties
Molecular Formula |
C5H10N2O2 |
---|---|
Molecular Weight |
130.15 g/mol |
IUPAC Name |
(3R)-morpholine-3-carboxamide |
InChI |
InChI=1S/C5H10N2O2/c6-5(8)4-3-9-2-1-7-4/h4,7H,1-3H2,(H2,6,8)/t4-/m1/s1 |
InChI Key |
YPSPPJRTCRMQGD-SCSAIBSYSA-N |
Isomeric SMILES |
C1COC[C@@H](N1)C(=O)N |
Canonical SMILES |
C1COCC(N1)C(=O)N |
Origin of Product |
United States |
Disclaimer and Information on In-Vitro Research Products
Please be aware that all articles and product information presented on BenchChem are intended solely for informational purposes. The products available for purchase on BenchChem are specifically designed for in-vitro studies, which are conducted outside of living organisms. In-vitro studies, derived from the Latin term "in glass," involve experiments performed in controlled laboratory settings using cells or tissues. It is important to note that these products are not categorized as medicines or drugs, and they have not received approval from the FDA for the prevention, treatment, or cure of any medical condition, ailment, or disease. We must emphasize that any form of bodily introduction of these products into humans or animals is strictly prohibited by law. It is essential to adhere to these guidelines to ensure compliance with legal and ethical standards in research and experimentation.