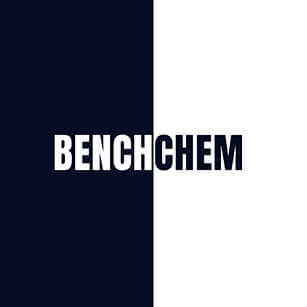
4-Methoxyphenol
- Click on QUICK INQUIRY to receive a quote from our team of experts.
- With the quality product at a COMPETITIVE price, you can focus more on your research.
Overview
Description
4-Methoxyphenol, also known as p-cresol or hydroquinone monomethyl ether, is an organic compound with the molecular formula C7H8O2. It is a phenolic compound characterized by a methoxy group attached to the para position of the benzene ring. This compound is widely used in various industries, including pharmaceuticals, cosmetics, and food packaging, due to its antioxidant and stabilizing properties .
Preparation Methods
Synthetic Routes and Reaction Conditions: 4-Methoxyphenol can be synthesized through several methods:
Methylation of Hydroquinone: One common method involves the methylation of hydroquinone using dimethyl sulfate or methanol under high temperature and pressure conditions.
Reaction with p-Benzoquinone: Another method involves the reaction of p-benzoquinone with methanol via a free radical mechanism.
Industrial Production Methods: In industrial settings, this compound is often produced by the methylation of hydroquinone using methanol in the presence of a catalyst. This method is preferred due to its efficiency and cost-effectiveness .
Chemical Reactions Analysis
4-Methoxyphenol undergoes various chemical reactions, including:
Reduction: Reduction reactions can convert this compound to its corresponding hydroquinone derivative.
Substitution: It can undergo electrophilic aromatic substitution reactions, such as nitration and halogenation, to form substituted derivatives.
Common Reagents and Conditions:
Oxidation: Common oxidizing agents include potassium permanganate and hydrogen peroxide.
Reduction: Reducing agents such as sodium borohydride and lithium aluminum hydride are often used.
Substitution: Reagents like nitric acid for nitration and halogens for halogenation are commonly employed.
Major Products:
Oxidation: Quinones
Reduction: Hydroquinone derivatives
Substitution: Substituted phenols
Scientific Research Applications
4-Methoxyphenol has a wide range of applications in scientific research:
Mechanism of Action
4-Methoxyphenol can be compared with other methoxyphenol isomers:
2-Methoxyphenol (Guaiacol): Used in the production of vanillin and as a precursor to green fuels.
3-Methoxyphenol: Less commonly used but has applications in organic synthesis.
Uniqueness: this compound is unique due to its dual role as an antioxidant and a polymerization inhibitor, making it valuable in both industrial and pharmaceutical applications .
Comparison with Similar Compounds
- 2-Methoxyphenol (Guaiacol)
- 3-Methoxyphenol
- Hydroquinone
Properties
CAS No. |
151-76-5 |
---|---|
Molecular Formula |
C11H16N2O3 |
Molecular Weight |
0 |
Origin of Product |
United States |
Disclaimer and Information on In-Vitro Research Products
Please be aware that all articles and product information presented on BenchChem are intended solely for informational purposes. The products available for purchase on BenchChem are specifically designed for in-vitro studies, which are conducted outside of living organisms. In-vitro studies, derived from the Latin term "in glass," involve experiments performed in controlled laboratory settings using cells or tissues. It is important to note that these products are not categorized as medicines or drugs, and they have not received approval from the FDA for the prevention, treatment, or cure of any medical condition, ailment, or disease. We must emphasize that any form of bodily introduction of these products into humans or animals is strictly prohibited by law. It is essential to adhere to these guidelines to ensure compliance with legal and ethical standards in research and experimentation.