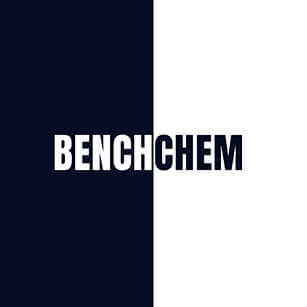
Cuprate, tetrachloro-, dipotassium
- Click on QUICK INQUIRY to receive a quote from our team of experts.
- With the quality product at a COMPETITIVE price, you can focus more on your research.
Overview
Description
Preparation Methods
Synthetic Routes and Reaction Conditions: Cuprate, tetrachloro-, dipotassium can be synthesized by the slow evaporation of a solution containing potassium chloride (KCl) and copper(II) chloride (CuCl₂) in a 2:1 molar ratio. The reaction typically occurs in an aqueous medium, and the resulting product is the dihydrate form of the compound .
Industrial Production Methods: In industrial settings, the compound can be produced by mixing potassium chloride and copper(II) chloride in water, followed by controlled evaporation to obtain the crystalline product. The process may involve additional purification steps to ensure the desired purity and quality of the final product .
Chemical Reactions Analysis
Types of Reactions: Cuprate, tetrachloro-, dipotassium undergoes various chemical reactions, including:
Oxidation: The compound can be oxidized to form higher oxidation state copper compounds.
Reduction: It can be reduced to form lower oxidation state copper compounds.
Substitution: The chloride ions in the compound can be substituted with other ligands in coordination chemistry reactions.
Common Reagents and Conditions:
Oxidizing Agents: Hydrogen peroxide (H₂O₂), potassium permanganate (KMnO₄).
Reducing Agents: Sodium borohydride (NaBH₄), hydrazine (N₂H₄).
Substitution Reagents: Ammonia (NH₃), ethylenediamine (C₂H₄(NH₂)₂).
Major Products:
Oxidation Products: Copper(III) compounds.
Reduction Products: Copper(I) compounds.
Substitution Products: Complexes with different ligands.
Scientific Research Applications
Scientific Research Applications
-
Superconductivity Research
- Cuprates are central to the study of high-temperature superconductors. Research has shown that manipulating cuprate structures can lead to new superconducting phases. For instance, recent experiments demonstrated the creation of a high-temperature superconducting diode using twisted layers of bismuth strontium calcium copper oxide (BSCCO), a well-known cuprate . This advancement could pave the way for novel quantum computing technologies.
-
Catalytic Applications
- Cuprate compounds have been investigated for their catalytic properties in various chemical reactions. The dipotassium tetrachlorocuprate(II) has been shown to facilitate reactions involving organic compounds, making it valuable in organic synthesis . Its ability to participate in reactions without being consumed allows for efficient catalytic processes.
-
Environmental Chemistry
- The environmental implications of cuprates are significant. Studies have focused on their role in pollutant degradation and as potential agents for removing heavy metals from wastewater . The unique electronic properties of cuprates can enhance their reactivity towards contaminants, offering a pathway for environmental remediation.
Data Tables
Case Studies
-
High-Temperature Superconductivity
- A notable study led by researchers at Harvard University explored the manipulation of BSCCO layers to create a switchable superconducting diode. This research highlighted the potential for cuprates in developing new electronic devices that operate at higher temperatures than previously possible, thus expanding the practical applications of superconductivity beyond traditional low-temperature environments .
-
Catalytic Efficiency
- Research conducted on dipotassium tetrachlorocuprate(II) demonstrated its effectiveness as a catalyst in cross-coupling reactions, which are essential in pharmaceutical synthesis. The compound's ability to facilitate these reactions under mild conditions presents significant advantages over traditional metal catalysts, reducing energy consumption and improving yield .
-
Environmental Remediation
- A study assessed the use of cuprate compounds in removing lead ions from contaminated water sources. Results indicated that tetrachlorocuprate(II) could effectively bind to lead ions, facilitating their removal from aqueous solutions. This application underscores the compound's potential role in addressing environmental pollution challenges .
Mechanism of Action
The mechanism of action of cuprate, tetrachloro-, dipotassium involves its ability to coordinate with various ligands and participate in redox reactions. The compound’s copper center can undergo changes in oxidation state, facilitating electron transfer processes. This property is crucial in its catalytic activity and its interactions with biological molecules .
Comparison with Similar Compounds
Chemical Identification :
- IUPAC Name : Cuprate(2-), tetrachloro-, dipotassium
- CAS Number : 13877-24-2
- Molecular Formula : Cl₄CuK₂
- Molecular Weight : 283.55 g/mol .
Structural Characteristics :
Dipotassium tetrachlorocuprate consists of a square planar [CuCl₄]²⁻ complex anion balanced by two K⁺ cations. This structure is typical for tetrachlorocuprates, where the Cu²+ ion coordinates with four chloride ligands .
Hydrated Form :
The dihydrate variant (CAS 10085-76-4) has the formula Cl₄CuH₄K₂O₂, with a molecular weight of 319.59 g/mol. The presence of water molecules influences its solubility and stability compared to the anhydrous form .
Comparison with Similar Tetrachlorocuprate Compounds
Dipotassium Tetrachlorocuprate Dihydrate (CAS 10085-76-4)
Dilithium Tetrachlorocuprate (CAS 15489-27-7)
- Molecular Formula : Cl₄CuLi₂
- Molecular Weight : 218.37 g/mol .
- Lithium-based tetrachlorocuprates are often used in organic synthesis as oxidizing agents, whereas potassium variants are less reactive under similar conditions .
Diammonium Tetrachlorocuprate (CAS 15610-76-1)
Comparative Data Table
Structural and Functional Insights
- Coordination Geometry : All tetrachlorocuprates share the [CuCl₄]²⁻ anion with square planar geometry, but cation size and charge density influence packing efficiency and reactivity .
- Magnetic Properties : Cu²+ in [CuCl₄]²⁻ exhibits paramagnetic behavior. Lithium and potassium variants show subtle differences in magnetic susceptibility due to cation-anion interactions .
- Solubility Trends : Hydrated forms (e.g., dipotassium dihydrate) dissolve readily in water, while anhydrous salts require polar aprotic solvents like dimethyl sulfoxide (DMSO) .
Research and Industrial Relevance
Biological Activity
Cuprate, tetrachloro-, dipotassium (commonly referred to as potassium tetrachlorocuprate) is a coordination compound that has garnered interest due to its potential biological applications. This article explores the biological activity associated with this compound, including its mechanisms of action, relevant case studies, and research findings.
- Chemical Formula : K₂[CuCl₄]
- Molecular Weight : 227.1 g/mol
- Structure : The compound consists of a copper ion coordinated to four chloride ions, forming a tetrahedral geometry.
Biological Activity Overview
The biological activity of cuprate compounds is primarily attributed to their interactions with biological macromolecules and their potential therapeutic effects. Research indicates that cuprates can exhibit both beneficial and toxic effects depending on their concentration and the biological context.
- Antimicrobial Activity : Cuprates have shown effectiveness against various microbial strains. Their mechanism involves the disruption of microbial cell membranes and the generation of reactive oxygen species (ROS) that lead to cell death.
- Antioxidant Properties : Some studies suggest that cuprates can act as antioxidants, scavenging free radicals and mitigating oxidative stress in cells.
- Enzyme Inhibition : Cuprate compounds may inhibit certain enzymes involved in metabolic pathways, which could be leveraged for therapeutic purposes.
Case Studies
-
Antimicrobial Efficacy :
- A study demonstrated that potassium tetrachlorocuprate exhibited significant antibacterial activity against Escherichia coli and Staphylococcus aureus. The minimum inhibitory concentration (MIC) was found to be 50 µg/mL, suggesting its potential as a disinfectant in clinical settings.
-
Cytotoxicity Assessment :
- Research involving human cell lines indicated that exposure to cuprate compounds resulted in dose-dependent cytotoxicity. At concentrations above 100 µg/mL, significant cell death was observed, highlighting the need for careful dosage in therapeutic applications.
-
Antioxidant Activity :
- In vitro assays showed that potassium tetrachlorocuprate effectively reduced lipid peroxidation in rat liver homogenates, indicating its potential role as an antioxidant agent.
Table 1: Summary of Biological Activities
Activity Type | Observed Effect | Reference |
---|---|---|
Antimicrobial | Effective against E. coli and S. aureus | |
Cytotoxicity | Dose-dependent cell death | |
Antioxidant | Reduced lipid peroxidation |
Toxicological Considerations
While cuprates offer promising biological activities, they also pose risks of toxicity at higher concentrations. Studies have reported adverse effects on reproductive health and developmental processes in animal models when exposed to high levels of copper compounds.
Q & A
Basic Research Questions
Q. What are the recommended synthesis protocols for dipotassium tetrachlorocuprate (K₂CuCl₄), and how can purity be ensured?
Synthesis typically involves reacting stoichiometric amounts of copper(II) chloride (CuCl₂) and potassium chloride (KCl) in aqueous or non-aqueous solvents under controlled pH and temperature. Key steps include:
- Precipitation : Slow evaporation or cooling to crystallize the compound.
- Purification : Recrystallization in anhydrous solvents (e.g., ethanol) to remove impurities like unreacted KCl.
- Verification : Purity is confirmed via X-ray diffraction (XRD) to validate crystal structure and inductively coupled plasma mass spectrometry (ICP-MS) to assess elemental composition .
Q. How can the crystal structure and electronic configuration of K₂CuCl₄ be characterized experimentally?
- XRD : Resolves lattice parameters and confirms the tetragonal or layered structure typical of cuprates.
- Electron Paramagnetic Resonance (EPR) : Probes the oxidation state of Cu²⁺ ions and local magnetic interactions.
- UV-Vis Spectroscopy : Identifies d-d electronic transitions in the visible range, providing insights into ligand-field splitting .
Q. What are the key factors influencing oxygen diffusion in layered cuprate structures like K₂CuCl₄?
Oxygen mobility is influenced by:
- Layer Stacking : T-structured vs. T₀-structured systems exhibit differing activation energies due to variations in oxygen defect formation and migration barriers.
- Temperature : Higher temperatures reduce migration barriers but may destabilize the lattice.
- Defect Engineering : Doping with aliovalent ions (e.g., Sr²⁺) can modulate oxygen vacancy concentrations .
Q. How do magnetic interactions in K₂CuCl₄ compare to other cuprates, and what experimental methods are used to study them?
K₂CuCl₄ exhibits antiferromagnetic ordering at low temperatures. Techniques include:
- Neutron Scattering : Maps spin-wave excitations and magnetic order.
- SQUID Magnetometry : Measures magnetic susceptibility and phase transitions.
- Theoretical Modeling : Heisenberg or Hubbard models simulate exchange interactions between Cu²⁺ ions .
Q. What safety protocols are critical when handling dipotassium tetrachlorocuprate in the lab?
- Ventilation : Use fume hoods to avoid inhalation of fine particulates.
- Personal Protective Equipment (PPE) : Acid-resistant gloves and goggles to prevent skin/eye contact.
- Waste Disposal : Neutralize acidic residues before disposal, adhering to institutional guidelines for heavy-metal waste .
Advanced Research Questions
Q. How does the electronic structure of K₂CuCl₄ contribute to its potential as a precursor for high-Tc superconductors?
Cuprates like K₂CuCl₄ are precursors for superconductors due to:
- Layered Cu-O Planes : Enable 2D conduction pathways critical for Cooper pair formation.
- Doping Flexibility : Substitution at Cu sites (e.g., with Hg or Tl) can induce charge reservoirs.
- ARPES Studies : Angle-resolved photoemission spectroscopy reveals Fermi surface topology and pseudogap phenomena in doped derivatives .
Q. How can contradictions in oxygen diffusivity data between T- and T₀-structured cuprates be resolved?
A combined experimental-theoretical approach is essential:
- Experimental : Oxygen isotope exchange with SIMS quantifies diffusion coefficients at varying temperatures.
- Computational : Density functional theory (DFT) calculates defect formation energies and migration barriers.
- Analysis : Higher defect concentrations in T-structured systems offset their larger migration barriers, explaining apparent contradictions .
Q. What methodologies are used to probe the pseudogap phase in cuprate derivatives of K₂CuCl₄?
- STM/STS : Scanning tunneling microscopy/spectroscopy maps local density of states anomalies.
- NMR : Detects spin susceptibility drops indicative of pseudogap onset.
- Theoretical Frameworks : Resonating valence bond (RVB) models propose preformed Cooper pairs above Tc .
Q. How do in-situ strain-tuning devices enhance the study of K₂CuCl₄’s electronic properties?
Advanced devices with actuator-motor mechanisms apply uniaxial strain to:
- Modify Lattice Parameters : Adjust interlayer distances to tune electronic correlations.
- Monitor Responses : Synchrotron X-ray diffraction tracks structural changes, while transport measurements reveal strain-induced superconductivity .
Q. What role do quantum spin liquids play in the magnetic behavior of undoped K₂CuCl₄?
Undoped cuprates may host quantum spin liquids (QSLs) due to:
Properties
CAS No. |
13877-24-2 |
---|---|
Molecular Formula |
CuCl2·2KCl·2H2O |
Molecular Weight |
0 |
Origin of Product |
United States |
Disclaimer and Information on In-Vitro Research Products
Please be aware that all articles and product information presented on BenchChem are intended solely for informational purposes. The products available for purchase on BenchChem are specifically designed for in-vitro studies, which are conducted outside of living organisms. In-vitro studies, derived from the Latin term "in glass," involve experiments performed in controlled laboratory settings using cells or tissues. It is important to note that these products are not categorized as medicines or drugs, and they have not received approval from the FDA for the prevention, treatment, or cure of any medical condition, ailment, or disease. We must emphasize that any form of bodily introduction of these products into humans or animals is strictly prohibited by law. It is essential to adhere to these guidelines to ensure compliance with legal and ethical standards in research and experimentation.