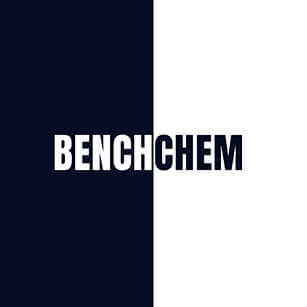
DIRECT BLUE 200
- Click on QUICK INQUIRY to receive a quote from our team of experts.
- With the quality product at a COMPETITIVE price, you can focus more on your research.
Overview
Description
DIRECT BLUE 200, also known as C.I. This compound, is an organic dye widely used in various industries. It is a dark blue powder that is soluble in water, alcohol, and other organic solvents. The chemical structure of this compound contains blue aromatic oligomeric benzene ring structures, which contribute to its vibrant color. This dye is primarily used in the textile, leather, and paper industries for dyeing purposes .
Preparation Methods
The synthesis of DIRECT BLUE 200 involves several chemical reactions, including dimerization and nitration of aniline. The industrial production of this dye typically follows these steps:
Dimerization Reaction: Aniline undergoes a dimerization reaction to form a dimeric intermediate.
Nitration Reaction: The dimeric intermediate is then subjected to nitration, resulting in the formation of this compound.
Chemical Reactions Analysis
DIRECT BLUE 200 undergoes various chemical reactions, including:
Oxidation: The dye can be oxidized under specific conditions, leading to the formation of different oxidation products.
Reduction: Reduction reactions can break down the dye into simpler compounds.
Substitution: The aromatic rings in this compound can undergo substitution reactions with various reagents.
Common reagents used in these reactions include oxidizing agents like hydrogen peroxide and reducing agents like sodium dithionite. The major products formed from these reactions depend on the specific conditions and reagents used .
Scientific Research Applications
DIRECT BLUE 200 has several scientific research applications, including:
Chemistry: It is used as a fluorescent probe for analyzing DNA and labeling agents in fluorescence microscopy.
Biology: The dye is employed in various biological staining techniques to visualize cellular components.
Medicine: this compound is used in biomedical research for studying cellular processes and interactions.
Industry: It is widely used in the textile industry for dyeing cotton, viscose, and other fabrics. .
Mechanism of Action
The mechanism of action of DIRECT BLUE 200 involves its interaction with molecular targets, primarily through its aromatic ring structures. These interactions can lead to changes in the physical and chemical properties of the target molecules. The dye’s ability to absorb and emit light makes it useful in fluorescence-based applications, where it can label and track specific molecules within biological systems .
Comparison with Similar Compounds
DIRECT BLUE 200 is part of the direct dye family, which includes other compounds such as C.I. Direct Blue 1 and C.I. Direct Orange 25. Compared to these similar compounds, this compound is unique due to its specific chemical structure and the vibrant blue color it imparts. Other similar compounds include:
C.I. Direct Blue 1: Known for its use in dyeing cotton and other cellulosic materials.
C.I. Direct Orange 25: Used for dyeing textiles and has different chromophore groups compared to this compound
This compound stands out due to its specific applications in fluorescence microscopy and its unique chemical interactions.
Properties
CAS No. |
12217-57-1 |
---|---|
Molecular Formula |
C15H33N3 |
Molecular Weight |
0 |
Origin of Product |
United States |
Disclaimer and Information on In-Vitro Research Products
Please be aware that all articles and product information presented on BenchChem are intended solely for informational purposes. The products available for purchase on BenchChem are specifically designed for in-vitro studies, which are conducted outside of living organisms. In-vitro studies, derived from the Latin term "in glass," involve experiments performed in controlled laboratory settings using cells or tissues. It is important to note that these products are not categorized as medicines or drugs, and they have not received approval from the FDA for the prevention, treatment, or cure of any medical condition, ailment, or disease. We must emphasize that any form of bodily introduction of these products into humans or animals is strictly prohibited by law. It is essential to adhere to these guidelines to ensure compliance with legal and ethical standards in research and experimentation.