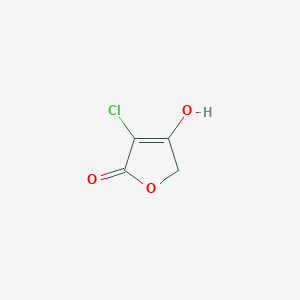
2(5H)-Furanone, 3-chloro-4-hydroxy-(9CI)
- Click on QUICK INQUIRY to receive a quote from our team of experts.
- With the quality product at a COMPETITIVE price, you can focus more on your research.
Overview
Description
2(5H)-Furanone, 3-chloro-4-hydroxy-(9CI) is a chemical compound with the molecular formula C4H3ClO3. It belongs to the class of β-hydroxybutenolides, which are known for their diverse biological properties. This compound is characterized by the presence of a furanone ring with a chlorine atom at the third position and a hydroxyl group at the fourth position .
Preparation Methods
Synthetic Routes and Reaction Conditions
The synthesis of 2(5H)-Furanone, 3-chloro-4-hydroxy-(9CI) typically involves the chlorination of 4-hydroxy-2(5H)-furanone. One common method is the reaction of 4-hydroxy-2(5H)-furanone with thionyl chloride (SOCl2) under controlled conditions. The reaction is carried out in an inert solvent such as dichloromethane at low temperatures to prevent decomposition .
Industrial Production Methods
Industrial production of this compound may involve continuous flow processes to ensure consistent quality and yield. The use of automated systems allows for precise control of reaction parameters, such as temperature, pressure, and reactant concentrations, which are crucial for the efficient production of 2(5H)-Furanone, 3-chloro-4-hydroxy-(9CI) .
Chemical Reactions Analysis
Types of Reactions
2(5H)-Furanone, 3-chloro-4-hydroxy-(9CI) undergoes various chemical reactions, including:
Oxidation: The hydroxyl group can be oxidized to form a carbonyl group.
Reduction: The compound can be reduced to form 3-chloro-4-hydroxybutanoic acid.
Substitution: The chlorine atom can be substituted with other nucleophiles, such as amines or thiols.
Common Reagents and Conditions
Oxidation: Common oxidizing agents include potassium permanganate (KMnO4) and chromium trioxide (CrO3).
Reduction: Reducing agents such as sodium borohydride (NaBH4) or lithium aluminum hydride (LiAlH4) are used.
Substitution: Nucleophilic substitution reactions often use reagents like sodium azide (NaN3) or thiourea (NH2CSNH2).
Major Products
Oxidation: 3-chloro-4-oxofuran-2(5H)-one.
Reduction: 3-chloro-4-hydroxybutanoic acid.
Substitution: Various substituted furanones depending on the nucleophile used.
Scientific Research Applications
2(5H)-Furanone, 3-chloro-4-hydroxy-(9CI) has several applications in scientific research:
Chemistry: Used as an intermediate in the synthesis of more complex organic molecules.
Biology: Studied for its potential antimicrobial and antifungal properties.
Medicine: Investigated for its potential use in drug development, particularly in the synthesis of pharmacologically active compounds.
Industry: Utilized in the production of specialty chemicals and as a building block in organic synthesis
Mechanism of Action
The mechanism of action of 2(5H)-Furanone, 3-chloro-4-hydroxy-(9CI) involves its interaction with various molecular targets. The hydroxyl group can form hydrogen bonds with biological molecules, while the chlorine atom can participate in halogen bonding. These interactions can affect the compound’s binding affinity and specificity towards enzymes and receptors, influencing its biological activity .
Comparison with Similar Compounds
Similar Compounds
4-Hydroxy-2(5H)-furanone: Lacks the chlorine atom, making it less reactive in certain substitution reactions.
3-Chloro-4-hydroxy-2-piperidone: Contains a piperidone ring instead of a furanone ring, leading to different chemical properties and reactivity.
Uniqueness
2(5H)-Furanone, 3-chloro-4-hydroxy-(9CI) is unique due to the presence of both a chlorine atom and a hydroxyl group on the furanone ring. This combination allows for a wide range of chemical reactions and interactions, making it a versatile compound in various fields of research .
Properties
Molecular Formula |
C4H3ClO3 |
---|---|
Molecular Weight |
134.52 g/mol |
IUPAC Name |
4-chloro-3-hydroxy-2H-furan-5-one |
InChI |
InChI=1S/C4H3ClO3/c5-3-2(6)1-8-4(3)7/h6H,1H2 |
InChI Key |
XKOKWDCFCYFJTJ-UHFFFAOYSA-N |
Canonical SMILES |
C1C(=C(C(=O)O1)Cl)O |
Origin of Product |
United States |
Disclaimer and Information on In-Vitro Research Products
Please be aware that all articles and product information presented on BenchChem are intended solely for informational purposes. The products available for purchase on BenchChem are specifically designed for in-vitro studies, which are conducted outside of living organisms. In-vitro studies, derived from the Latin term "in glass," involve experiments performed in controlled laboratory settings using cells or tissues. It is important to note that these products are not categorized as medicines or drugs, and they have not received approval from the FDA for the prevention, treatment, or cure of any medical condition, ailment, or disease. We must emphasize that any form of bodily introduction of these products into humans or animals is strictly prohibited by law. It is essential to adhere to these guidelines to ensure compliance with legal and ethical standards in research and experimentation.