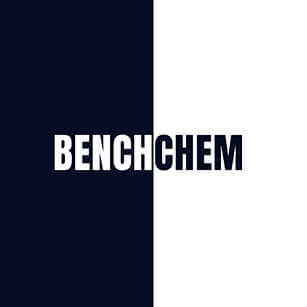
Gallium(III) hydride.
Overview
Description
Gallium(III) hydride is an inorganic compound with the chemical formula GaH3 It is a hydride of gallium, where gallium is in the +3 oxidation state
Preparation Methods
Synthetic Routes and Reaction Conditions: Gallium(III) hydride can be synthesized through several methods. One common approach involves the reduction of gallium(III) chloride with lithium aluminum hydride. The reaction typically occurs under an inert atmosphere to prevent oxidation:
GaCl3+LiAlH4→GaH3+LiCl+AlCl3
Industrial Production Methods: Industrial production of gallium(III) hydride is less common due to its instability and reactivity. advancements in synthetic techniques and stabilization methods may pave the way for its large-scale production in the future.
Chemical Reactions Analysis
Types of Reactions: Gallium(III) hydride undergoes various chemical reactions, including:
Oxidation: Gallium(III) hydride can be oxidized to form gallium oxide.
Reduction: It can be reduced to elemental gallium.
Substitution: Gallium(III) hydride can participate in substitution reactions with other hydrides or halides.
Common Reagents and Conditions:
Oxidation: Oxygen or other oxidizing agents.
Reduction: Strong reducing agents like lithium aluminum hydride.
Substitution: Halides or other hydrides under controlled conditions.
Major Products Formed:
Oxidation: Gallium oxide (Ga2O3).
Reduction: Elemental gallium (Ga).
Substitution: Various gallium-containing compounds depending on the substituent.
Scientific Research Applications
Gallium(III) hydride has several scientific research applications:
Catalysis: It is used as a catalyst in hydrogenation reactions and other organic transformations.
Hydrogen Storage: Due to its high hydrogen content, it is explored as a potential hydrogen storage material.
Electronics: Gallium compounds are used in semiconductors and other electronic devices.
Medical Applications: Gallium compounds have shown potential in antimicrobial and anticancer therapies.
Mechanism of Action
The mechanism of action of gallium(III) hydride involves its ability to donate hydride ions (H-) in chemical reactions. This property makes it a valuable reducing agent and catalyst. The molecular targets and pathways involved depend on the specific application, such as hydrogenation or reduction reactions .
Comparison with Similar Compounds
Diborane (B2H6): Similar in structure and reactivity, used in hydrogenation and as a reducing agent.
Aluminum Hydride (AlH3): Another group 13 hydride with similar applications in hydrogen storage and reduction reactions.
Indium Hydride (InH3): Less common but shares similar chemical properties with gallium(III) hydride.
Uniqueness: Its ability to form stable complexes with various ligands also sets it apart from other hydrides .
Properties
IUPAC Name |
gallane | |
---|---|---|
Details | Computed by Lexichem TK 2.7.0 (PubChem release 2021.05.07) | |
Source | PubChem | |
URL | https://pubchem.ncbi.nlm.nih.gov | |
Description | Data deposited in or computed by PubChem | |
InChI |
InChI=1S/Ga.3H | |
Details | Computed by InChI 1.0.6 (PubChem release 2021.05.07) | |
Source | PubChem | |
URL | https://pubchem.ncbi.nlm.nih.gov | |
Description | Data deposited in or computed by PubChem | |
InChI Key |
PHMDYZQXPPOZDG-UHFFFAOYSA-N | |
Details | Computed by InChI 1.0.6 (PubChem release 2021.05.07) | |
Source | PubChem | |
URL | https://pubchem.ncbi.nlm.nih.gov | |
Description | Data deposited in or computed by PubChem | |
Canonical SMILES |
[GaH3] | |
Details | Computed by OEChem 2.3.0 (PubChem release 2021.05.07) | |
Source | PubChem | |
URL | https://pubchem.ncbi.nlm.nih.gov | |
Description | Data deposited in or computed by PubChem | |
Molecular Formula |
GaH3 | |
Details | Computed by PubChem 2.1 (PubChem release 2021.05.07) | |
Source | PubChem | |
URL | https://pubchem.ncbi.nlm.nih.gov | |
Description | Data deposited in or computed by PubChem | |
DSSTOX Substance ID |
DTXSID8064696 | |
Record name | Gallium trihydride | |
Source | EPA DSSTox | |
URL | https://comptox.epa.gov/dashboard/DTXSID8064696 | |
Description | DSSTox provides a high quality public chemistry resource for supporting improved predictive toxicology. | |
Molecular Weight |
72.747 g/mol | |
Details | Computed by PubChem 2.1 (PubChem release 2021.08.13) | |
Source | PubChem | |
URL | https://pubchem.ncbi.nlm.nih.gov | |
Description | Data deposited in or computed by PubChem | |
Physical Description |
Liquid | |
Record name | Gallium | |
Source | Human Metabolome Database (HMDB) | |
URL | http://www.hmdb.ca/metabolites/HMDB0001478 | |
Description | The Human Metabolome Database (HMDB) is a freely available electronic database containing detailed information about small molecule metabolites found in the human body. | |
Explanation | HMDB is offered to the public as a freely available resource. Use and re-distribution of the data, in whole or in part, for commercial purposes requires explicit permission of the authors and explicit acknowledgment of the source material (HMDB) and the original publication (see the HMDB citing page). We ask that users who download significant portions of the database cite the HMDB paper in any resulting publications. | |
CAS No. |
13572-93-5, 7440-55-3 | |
Record name | Gallium hydride (GaH3) | |
Source | CAS Common Chemistry | |
URL | https://commonchemistry.cas.org/detail?cas_rn=13572-93-5 | |
Description | CAS Common Chemistry is an open community resource for accessing chemical information. Nearly 500,000 chemical substances from CAS REGISTRY cover areas of community interest, including common and frequently regulated chemicals, and those relevant to high school and undergraduate chemistry classes. This chemical information, curated by our expert scientists, is provided in alignment with our mission as a division of the American Chemical Society. | |
Explanation | The data from CAS Common Chemistry is provided under a CC-BY-NC 4.0 license, unless otherwise stated. | |
Record name | Gallium trihydride | |
Source | EPA DSSTox | |
URL | https://comptox.epa.gov/dashboard/DTXSID8064696 | |
Description | DSSTox provides a high quality public chemistry resource for supporting improved predictive toxicology. | |
Record name | Gallium | |
Source | Human Metabolome Database (HMDB) | |
URL | http://www.hmdb.ca/metabolites/HMDB0001478 | |
Description | The Human Metabolome Database (HMDB) is a freely available electronic database containing detailed information about small molecule metabolites found in the human body. | |
Explanation | HMDB is offered to the public as a freely available resource. Use and re-distribution of the data, in whole or in part, for commercial purposes requires explicit permission of the authors and explicit acknowledgment of the source material (HMDB) and the original publication (see the HMDB citing page). We ask that users who download significant portions of the database cite the HMDB paper in any resulting publications. | |
Disclaimer and Information on In-Vitro Research Products
Please be aware that all articles and product information presented on BenchChem are intended solely for informational purposes. The products available for purchase on BenchChem are specifically designed for in-vitro studies, which are conducted outside of living organisms. In-vitro studies, derived from the Latin term "in glass," involve experiments performed in controlled laboratory settings using cells or tissues. It is important to note that these products are not categorized as medicines or drugs, and they have not received approval from the FDA for the prevention, treatment, or cure of any medical condition, ailment, or disease. We must emphasize that any form of bodily introduction of these products into humans or animals is strictly prohibited by law. It is essential to adhere to these guidelines to ensure compliance with legal and ethical standards in research and experimentation.