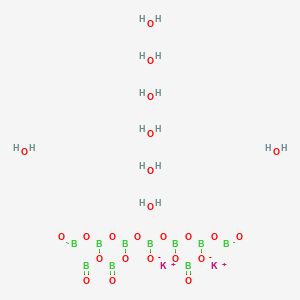
Potassium pentaborate octahydrate
Overview
Description
Potassium pentaborate octahydrate (K2B10O16·8H2O) is recognized for its utility as an additive in industrial and automotive lubricants. Its particle size is crucial for achieving a stable dispersion in high-pressure lubricant applications, making the understanding of its crystal growth kinetics essential for obtaining the desired particle size distribution.
Synthesis Analysis
The synthesis of potassium pentaborate involves various chemical reactions and processes. One study detailed the growth rate investigation of this compound in a fluidized bed crystallizer, emphasizing the need to understand crystal growth kinetics for desired particle size distribution in lubricant applications (Gürbüz, Badem, & Bulutcu, 2005).
Molecular Structure Analysis
The molecular structure of potassium pentaborate has been elucidated through various analytical techniques. One such study using neutron diffraction data analyzed the atomic coordinates, particularly of hydrogen atoms, in potassium pentaborate tetrahydrate, providing insights into the hydrogen positions and water molecule geometry (Ashmore & Petch, 1970).
Chemical Reactions and Properties
Chemical reactions involving potassium pentaborate are complex and multifaceted. For instance, the reaction dynamics and structural derivatization of potassium complexes in organofullerene chemistry have implications for understanding the chemical behavior and properties of potassium pentaborate-related compounds (Matsuo & Nakamura, 2005).
Physical Properties Analysis
The study of potassium pentaborate's physical properties includes investigations into its crystal growth, optical, mechanical, and dielectric properties. For example, research on the growth and properties of potassium pentaborate dihydrate crystals highlights their potential for various device applications due to their stability, mechanical strength, and dielectric behavior (Ramki & Vizhi, 2019).
Chemical Properties Analysis
The chemical properties of potassium pentaborate, such as its reactions with other compounds and behavior under various conditions, are crucial for its application in different fields. The synthesis and investigation of lithium octahydrotriborate, for example, offer insights into the thermal decomposition and potential use of related borohydride compounds, including those involving potassium pentaborate (Fu et al., 2016).
Scientific Research Applications
Industrial and Automotive Lubricants : Potassium pentaborate octahydrate is used as an additive in industrial and automotive lubricants. Its crystal growth kinetics are crucial for achieving a stable dispersion in high-pressure lubricants, as the particle size of crystals significantly impacts lubricant performance (Gürbüz, Badem, & Bulutcu, 2005).
Recovery of Potassium Salts : This compound is also involved in processes for recovering potassium content from bittern, a by-product of salt production. This compound can be crystallized from bittern by adding sodium pentaborate, and under optimal conditions, a significant percentage of potassium can be recovered (Gurbuz, Yavasoglu, & Bulutcu, 1996).
Optical and Non-linear Optical Properties : Studies have shown that compounds related to potassium pentaborate possess significant non-linear optical (NLO) properties, which are essential for various photonic and electro-optic applications. For example, a synthesized nonmetal pentaborate displayed second-harmonic generation efficiency close to that of potassium dihydrogen phosphate, a common NLO material (Liu, Liang, & Jiang, 2008).
Structural and Hydration Studies : Research into the structure of aqueous potassium pentaborate solution has provided insights into the hydration characteristics of potassium and borate ions. Such studies are significant for understanding the solution behavior of borate minerals and their applications in various chemical processes (Zhu et al., 2015).
Synthesis and Characterization : Potassium pentaborate can be synthesized from various materials and under different conditions. Its synthesis and characterization have implications for materials science, especially in creating compounds with specific physical properties for industrial applications (Çelik & Kurtuluş, 2011).
Safety and Hazards
Future Directions
Potassium pentaborate octahydrate has been used in the synthesis of L-Proline this compound single crystals, which have potential applications in the field of optoelectronics, optical communication, energy storage, and data storage . The semiorganic crystal has both organic and inorganic quality, making it a promising candidate for various linear and nonlinear applications .
Mechanism of Action
Target of Action
Potassium pentaborate octahydrate primarily targets the potassium channels in cells . These channels play a crucial role in maintaining the electrochemical gradients across the cell membrane, which are essential for normal cellular functions such as nerve transmission, muscle contraction, and kidney function .
Mode of Action
this compound interacts with its targets by inhibiting repolarizing potassium currents . This inhibition prolongs the action potential duration, leading to an increase in the effective refractory period (ERP) . The compound’s interaction with potassium channels can also lead to changes in the transmembrane electrochemical gradients .
Biochemical Pathways
The compound’s action affects the potassium homeostasis pathway . This pathway is responsible for maintaining the balance of potassium in the body. Alterations in this pathway can lead to conditions such as hypokalemia (low potassium) or hyperkalemia (high potassium) .
Pharmacokinetics
It is known that potassium is absorbed via passive diffusion, primarily in the small intestine . About 90% of ingested potassium is absorbed and used to maintain its normal intracellular and extracellular concentrations . Potassium is excreted primarily in the urine .
Result of Action
The molecular and cellular effects of this compound’s action include changes in the action potential duration and ERP . These changes can affect the excitability of cells, particularly neurons and muscle cells . Additionally, the compound’s action can lead to alterations in the transmembrane electrochemical gradients .
Action Environment
Environmental factors can influence the action, efficacy, and stability of this compound. For instance, the growth rates of this compound crystals can be influenced by an external potential . Additionally, the compound’s action can be affected by the intensity of mixing and cooling rates during crystallization .
properties
IUPAC Name |
dipotassium;[bis(oxoboranyloxy)boranyloxy-oxoboranyloxyboranyl]oxy-[[oxido(oxoboranyloxy)boranyl]oxy-oxoboranyloxyboranyl]oxyborinate;octahydrate | |
---|---|---|
Source | PubChem | |
URL | https://pubchem.ncbi.nlm.nih.gov | |
Description | Data deposited in or computed by PubChem | |
InChI |
InChI=1S/B10O16.2K.8H2O/c11-1-18-6(16)23-9(21-4-14)24-7(17)25-10(22-5-15)26-8(19-2-12)20-3-13;;;;;;;;;;/h;;;8*1H2/q-2;2*+1;;;;;;;; | |
Source | PubChem | |
URL | https://pubchem.ncbi.nlm.nih.gov | |
Description | Data deposited in or computed by PubChem | |
InChI Key |
AXTXIARPFKLBPW-UHFFFAOYSA-N | |
Source | PubChem | |
URL | https://pubchem.ncbi.nlm.nih.gov | |
Description | Data deposited in or computed by PubChem | |
Canonical SMILES |
B(=O)OB([O-])OB(OB=O)OB([O-])OB(OB=O)OB(OB=O)OB=O.O.O.O.O.O.O.O.O.[K+].[K+] | |
Source | PubChem | |
URL | https://pubchem.ncbi.nlm.nih.gov | |
Description | Data deposited in or computed by PubChem | |
Molecular Formula |
B10H16K2O24 | |
Source | PubChem | |
URL | https://pubchem.ncbi.nlm.nih.gov | |
Description | Data deposited in or computed by PubChem | |
Molecular Weight |
586.4 g/mol | |
Source | PubChem | |
URL | https://pubchem.ncbi.nlm.nih.gov | |
Description | Data deposited in or computed by PubChem | |
CAS RN |
12229-13-9 | |
Record name | Boron potassium oxide (B5KO8), tetrahydrate | |
Source | European Chemicals Agency (ECHA) | |
URL | https://echa.europa.eu/substance-information/-/substanceinfo/100.120.837 | |
Description | The European Chemicals Agency (ECHA) is an agency of the European Union which is the driving force among regulatory authorities in implementing the EU's groundbreaking chemicals legislation for the benefit of human health and the environment as well as for innovation and competitiveness. | |
Explanation | Use of the information, documents and data from the ECHA website is subject to the terms and conditions of this Legal Notice, and subject to other binding limitations provided for under applicable law, the information, documents and data made available on the ECHA website may be reproduced, distributed and/or used, totally or in part, for non-commercial purposes provided that ECHA is acknowledged as the source: "Source: European Chemicals Agency, http://echa.europa.eu/". Such acknowledgement must be included in each copy of the material. ECHA permits and encourages organisations and individuals to create links to the ECHA website under the following cumulative conditions: Links can only be made to webpages that provide a link to the Legal Notice page. | |
Record name | potassium pentaborate octahydrate | |
Source | European Chemicals Agency (ECHA) | |
URL | https://echa.europa.eu/information-on-chemicals | |
Description | The European Chemicals Agency (ECHA) is an agency of the European Union which is the driving force among regulatory authorities in implementing the EU's groundbreaking chemicals legislation for the benefit of human health and the environment as well as for innovation and competitiveness. | |
Explanation | Use of the information, documents and data from the ECHA website is subject to the terms and conditions of this Legal Notice, and subject to other binding limitations provided for under applicable law, the information, documents and data made available on the ECHA website may be reproduced, distributed and/or used, totally or in part, for non-commercial purposes provided that ECHA is acknowledged as the source: "Source: European Chemicals Agency, http://echa.europa.eu/". Such acknowledgement must be included in each copy of the material. ECHA permits and encourages organisations and individuals to create links to the ECHA website under the following cumulative conditions: Links can only be made to webpages that provide a link to the Legal Notice page. | |
Q & A
Q1: What are the optimal conditions for crystallizing potassium pentaborate octahydrate from bittern, and what is the potential yield?
A1: Research suggests that the optimal conditions for this compound crystallization from bittern are achieved at a temperature of 15°C using a sodium pentaborate solution pre-saturated at 25°C []. Under these conditions, approximately 65% of the potassium content in the bittern can be recovered as this compound crystals []. This method presents a promising avenue for potassium recovery from this industrial byproduct.
Q2: Besides its potential use in potassium recovery, are there other applications for this compound?
A2: Yes, recent research has explored the nonlinear optical properties of this compound []. Specifically, L-Proline this compound (LPPPB) single crystals have shown promise for optical limiting applications []. This highlights the material's potential in advanced optical technologies.
Q3: Is there any research available on alternative methods for producing this compound?
A3: While specific details are limited, a study mentions the determination of production conditions for this compound []. Further investigation into this research could reveal alternative synthesis routes and potentially lead to more efficient production methods.
Disclaimer and Information on In-Vitro Research Products
Please be aware that all articles and product information presented on BenchChem are intended solely for informational purposes. The products available for purchase on BenchChem are specifically designed for in-vitro studies, which are conducted outside of living organisms. In-vitro studies, derived from the Latin term "in glass," involve experiments performed in controlled laboratory settings using cells or tissues. It is important to note that these products are not categorized as medicines or drugs, and they have not received approval from the FDA for the prevention, treatment, or cure of any medical condition, ailment, or disease. We must emphasize that any form of bodily introduction of these products into humans or animals is strictly prohibited by law. It is essential to adhere to these guidelines to ensure compliance with legal and ethical standards in research and experimentation.