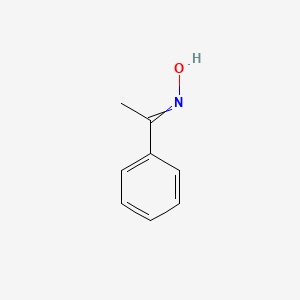
Acetophenone oxime
Overview
Description
Acetophenone oxime is an organic compound with the molecular formula C8H9NO. It is a derivative of acetophenone, where the carbonyl group is converted into an oxime group (-C=N-OH). This compound is commonly used in organic synthesis and has various applications in scientific research and industry.
Preparation Methods
Synthetic Routes and Reaction Conditions
Acetophenone oxime is typically synthesized by reacting acetophenone with hydroxylamine hydrochloride in the presence of a base such as sodium acetate and ethanol. The reaction involves the following steps :
- Dissolve acetophenone (1.2 g, 10 mmol) in 20 mL of ethanol in a 50 mL round-bottom flask.
- Add hydroxylamine hydrochloride (1.18 g, 17 mmol) and sodium acetate trihydrate (0.82 g, 10 mmol), which should already be dissolved in 15 mL of warm water, to the flask.
- Heat the mixture under reflux on a water bath for 20 minutes.
- Quickly filter the hot solution through a fluted filter paper.
- Cool the filtrate in an ice bath to collect the crystalline oxime (a white crystalline solid).
- Filter the crystals under vacuum, wash with a small volume of cold 50% ethanol, and dry them on the filter with suction.
Industrial Production Methods
Industrial production of this compound often involves similar synthetic routes but on a larger scale. The use of continuous flow reactors and automated systems ensures consistent product quality and higher yields. Additionally, the reaction conditions may be optimized to reduce reaction time and improve efficiency.
Chemical Reactions Analysis
Types of Reactions
Acetophenone oxime undergoes various chemical reactions, including:
Beckmann Rearrangement: This reaction converts this compound into N-phenylacetamide using acid catalysts such as sulfuric acid or zeolites.
Hydrolysis: This compound can be hydrolyzed back to acetophenone and hydroxylamine under acidic conditions.
Common Reagents and Conditions
Beckmann Rearrangement: Acid catalysts like sulfuric acid, zeolites, or silicalite-N are commonly used.
Hydrolysis: Moderately concentrated aqueous acids are used for hydrolysis.
Major Products Formed
Beckmann Rearrangement: N-phenylacetamide.
Hydrolysis: Acetophenone and hydroxylamine.
Scientific Research Applications
Acetophenone oxime has several applications in scientific research, including:
Organic Synthesis: It is used as an intermediate in the synthesis of various organic compounds.
Catalysis: This compound acts as a ligand in transition-metal complex catalyst chemistry.
Antioxidant: It functions as an antioxidant and radical scavenger in the textile, plastic, paint, detergent, and rubber industries.
Mechanism of Action
The mechanism of action of acetophenone oxime in the Beckmann rearrangement involves the N-protonation of the oxime group on the acid sites of the catalyst. This leads to the formation of a nitrilium ion, followed by solvolysis to an imidate and subsequent tautomerization to the amide . The molecular targets and pathways involved include the acid sites of zeolites or other catalysts, which facilitate the rearrangement reaction.
Comparison with Similar Compounds
Similar Compounds
Cyclohexanone oxime: Undergoes Beckmann rearrangement to form ε-caprolactam, a precursor for Nylon 6.
Cyclododecanone oxime: Converts to ω-laurolactam, used in the production of Nylon 12.
Uniqueness
Acetophenone oxime is unique due to its specific structure and reactivity, which allows it to form N-phenylacetamide through the Beckmann rearrangement. This distinguishes it from other oximes like cyclohexanone oxime and cyclododecanone oxime, which form different lactams used in polymer production .
Properties
IUPAC Name |
N-(1-phenylethylidene)hydroxylamine | |
---|---|---|
Source | PubChem | |
URL | https://pubchem.ncbi.nlm.nih.gov | |
Description | Data deposited in or computed by PubChem | |
InChI |
InChI=1S/C8H9NO/c1-7(9-10)8-5-3-2-4-6-8/h2-6,10H,1H3 | |
Source | PubChem | |
URL | https://pubchem.ncbi.nlm.nih.gov | |
Description | Data deposited in or computed by PubChem | |
InChI Key |
JHNRZXQVBKRYKN-UHFFFAOYSA-N | |
Source | PubChem | |
URL | https://pubchem.ncbi.nlm.nih.gov | |
Description | Data deposited in or computed by PubChem | |
Canonical SMILES |
CC(=NO)C1=CC=CC=C1 | |
Source | PubChem | |
URL | https://pubchem.ncbi.nlm.nih.gov | |
Description | Data deposited in or computed by PubChem | |
Molecular Formula |
C8H9NO | |
Source | PubChem | |
URL | https://pubchem.ncbi.nlm.nih.gov | |
Description | Data deposited in or computed by PubChem | |
Molecular Weight |
135.16 g/mol | |
Source | PubChem | |
URL | https://pubchem.ncbi.nlm.nih.gov | |
Description | Data deposited in or computed by PubChem | |
CAS No. |
613-91-2 | |
Record name | Acetophenonoxime | |
Source | CAS Common Chemistry | |
URL | https://commonchemistry.cas.org/detail?cas_rn=613-91-2 | |
Description | CAS Common Chemistry is an open community resource for accessing chemical information. Nearly 500,000 chemical substances from CAS REGISTRY cover areas of community interest, including common and frequently regulated chemicals, and those relevant to high school and undergraduate chemistry classes. This chemical information, curated by our expert scientists, is provided in alignment with our mission as a division of the American Chemical Society. | |
Explanation | The data from CAS Common Chemistry is provided under a CC-BY-NC 4.0 license, unless otherwise stated. | |
Record name | Ethanone, 1-phenyl-, oxime | |
Source | EPA Chemicals under the TSCA | |
URL | https://www.epa.gov/chemicals-under-tsca | |
Description | EPA Chemicals under the Toxic Substances Control Act (TSCA) collection contains information on chemicals and their regulations under TSCA, including non-confidential content from the TSCA Chemical Substance Inventory and Chemical Data Reporting. | |
Record name | Acetophenone oxime | |
Source | European Chemicals Agency (ECHA) | |
URL | https://echa.europa.eu/substance-information/-/substanceinfo/100.009.420 | |
Description | The European Chemicals Agency (ECHA) is an agency of the European Union which is the driving force among regulatory authorities in implementing the EU's groundbreaking chemicals legislation for the benefit of human health and the environment as well as for innovation and competitiveness. | |
Explanation | Use of the information, documents and data from the ECHA website is subject to the terms and conditions of this Legal Notice, and subject to other binding limitations provided for under applicable law, the information, documents and data made available on the ECHA website may be reproduced, distributed and/or used, totally or in part, for non-commercial purposes provided that ECHA is acknowledged as the source: "Source: European Chemicals Agency, http://echa.europa.eu/". Such acknowledgement must be included in each copy of the material. ECHA permits and encourages organisations and individuals to create links to the ECHA website under the following cumulative conditions: Links can only be made to webpages that provide a link to the Legal Notice page. | |
Synthesis routes and methods I
Procedure details
Synthesis routes and methods II
Procedure details
Synthesis routes and methods III
Procedure details
Q1: Why is Ethanone, 1-phenyl-, oxime relevant in the context of biomass pyrolysis?
A: While identified as a constituent in the pyrolysis products of both cotton stalk [] and Eucalyptus urograndis wood [], Ethanone, 1-phenyl-, oxime is present in relatively small amounts. Its significance lies not in its abundance but in its potential implications. This compound, alongside other volatiles, contributes to the overall profile of emissions from biomass pyrolysis. Understanding the composition of these emissions is crucial for assessing potential environmental impacts and exploring potential applications of the pyrolysis byproducts.
Disclaimer and Information on In-Vitro Research Products
Please be aware that all articles and product information presented on BenchChem are intended solely for informational purposes. The products available for purchase on BenchChem are specifically designed for in-vitro studies, which are conducted outside of living organisms. In-vitro studies, derived from the Latin term "in glass," involve experiments performed in controlled laboratory settings using cells or tissues. It is important to note that these products are not categorized as medicines or drugs, and they have not received approval from the FDA for the prevention, treatment, or cure of any medical condition, ailment, or disease. We must emphasize that any form of bodily introduction of these products into humans or animals is strictly prohibited by law. It is essential to adhere to these guidelines to ensure compliance with legal and ethical standards in research and experimentation.