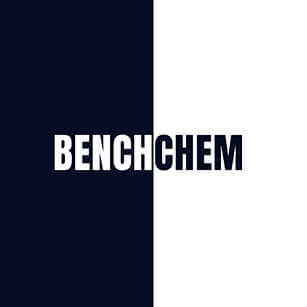
triphosphorus pentanitride
- Click on QUICK INQUIRY to receive a quote from our team of experts.
- With the quality product at a COMPETITIVE price, you can focus more on your research.
Overview
Description
Triphosphorus pentanitride is an inorganic compound with the chemical formula P₃N₅. It is classified as a binary nitride, containing only phosphorus and nitrogen. This compound is a white solid, although samples often appear colored due to impurities. Despite being investigated for various applications, this compound has not found significant industrial uses .
Preparation Methods
Synthetic Routes and Reaction Conditions: Triphosphorus pentanitride can be synthesized through several methods:
- Phosphorus pentachloride reacts with ammonia to produce this compound and hydrochloric acid:
Reaction with Ammonia: 3PCl5+5NH3→P3N5+15HCl
Phosphorus pentachloride reacts with sodium azide to yield this compound, sodium chloride, and nitrogen gas:Reaction with Sodium Azide: 3PCl5+15NaN3→P3N5+15NaCl+20N2
Hexachlorocyclotriphosphazene or phosphorus pentachloride reacts with ammonium chloride to form this compound and hydrochloric acid:Reaction with Ammonium Chloride: (NPCl2)3+2[NH4]Cl→P3N5+8HCl
3PCl5+5[NH4]Cl→P3N5+20HCl
Phosphorus trichloride reacts with sodium amide to produce this compound, sodium chloride, hydrochloric acid, and hydrogen gas:Reaction with Sodium Amide: 3PCl3+5NaNH2→P3N5+5NaCl+4HCl+3H2
Industrial Production Methods: Currently, there are no significant industrial production methods for this compound due to its limited commercial applications .
Types of Reactions:
- this compound decomposes to phosphorus mononitride and nitrogen gas at temperatures above 850°C:
Thermal Decomposition: P3N5→3PN+N2
Further decomposition of phosphorus mononitride yields elemental phosphorus and nitrogen gas:4PN→P4+2N2
Upon heating, this compound hydrolyzes to form ammonium phosphate salts:Hydrolysis: [NH4]2HPO4 and [NH4]H2PO4
It reacts with lithium nitride and calcium nitride to form corresponding salts of phosphorus nitride anions:Reactions with Metal Nitrides: PN74− and PN43−
Heterogeneous ammonolysis of this compound yields imides such as:Ammonolysis: HPN2 and HP4N7
Common Reagents and Conditions:
Reagents: Ammonia, sodium azide, ammonium chloride, sodium amide, lithium nitride, calcium nitride.
Conditions: High temperatures (above 850°C) for thermal decomposition, heating for hydrolysis, and specific stoichiometric ratios for reactions with metal nitrides and ammonolysis
Major Products:
Thermal Decomposition: Phosphorus mononitride, elemental phosphorus, nitrogen gas.
Hydrolysis: Ammonium phosphate salts.
Reactions with Metal Nitrides: Salts of phosphorus nitride anions.
Ammonolysis: Imides such as HPN₂ and HP₄N₇
Scientific Research Applications
Triphosphorus pentanitride has been explored for various scientific research applications, including:
Solid Electrolytes: Potential use in solid electrolytes due to its ionic conductivity.
Pigments: Investigated for use as pigments in various materials.
Gettering Material: Used as a gettering material in incandescent lamps, replacing mixtures containing red phosphorus
Mechanism of Action
The mechanism of action for triphosphorus pentanitride primarily involves its chemical reactivity with other compounds. It interacts with various reagents to form different products, as described in the chemical reactions analysis. The molecular targets and pathways involved depend on the specific reaction and the reagents used .
Comparison with Similar Compounds
Boron Nitride (BN): Similar in structure and synthesis methods but more thermally stable.
Silicon Nitride (Si₃N₄): Also similar in structure and synthesis methods, with higher thermal stability compared to triphosphorus pentanitride
Uniqueness:
Thermal Stability: this compound is less thermally stable than boron nitride and silicon nitride.
Applications: While boron nitride and silicon nitride have significant industrial applications, this compound’s applications are more limited and primarily in research
Properties
CAS No. |
12136-91-3 |
---|---|
Molecular Formula |
N5P3 |
Molecular Weight |
0 |
Synonyms |
Phosphorus pentanitride |
Origin of Product |
United States |
Disclaimer and Information on In-Vitro Research Products
Please be aware that all articles and product information presented on BenchChem are intended solely for informational purposes. The products available for purchase on BenchChem are specifically designed for in-vitro studies, which are conducted outside of living organisms. In-vitro studies, derived from the Latin term "in glass," involve experiments performed in controlled laboratory settings using cells or tissues. It is important to note that these products are not categorized as medicines or drugs, and they have not received approval from the FDA for the prevention, treatment, or cure of any medical condition, ailment, or disease. We must emphasize that any form of bodily introduction of these products into humans or animals is strictly prohibited by law. It is essential to adhere to these guidelines to ensure compliance with legal and ethical standards in research and experimentation.