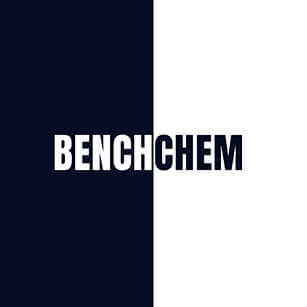
Gramicidin
- Click on QUICK INQUIRY to receive a quote from our team of experts.
- With the quality product at a COMPETITIVE price, you can focus more on your research.
Overview
Description
Gramicidin D is a bactericidal antibiotic composed of a mixture of three antibiotic compounds: gramicidins A, B, and C. These compounds are obtained from the soil bacterial species Bacillus brevis. This compound D is primarily used as a topical antibiotic due to its high hemolytic activity, which prevents its internal administration .
Mechanism of Action
Target of Action
Gramicidin, a potent antimicrobial peptide, primarily targets bacterial membranes . It exhibits high efficacy against most gram-positive bacteria and some gram-negative bacteria . The primary role of these targets is to maintain the integrity of the bacterial cell and regulate the transport of substances in and out of the cell.
Mode of Action
This compound interacts with its targets by binding to and inserting itself into bacterial membranes . It has a strong preference for gram-positive cell membranes . This interaction results in the disruption and permeabilization of the membrane, where this compound acts as a channel . This channel formation changes the ion gradient across the bacterial cell membrane .
Biochemical Pathways
The action of this compound affects several biochemical pathways. It disrupts the ion concentration gradients of the plasma membrane and also localizes in the mitochondria, depolarizing the inner mitochondrial membrane . The diminished H+ gradient in the mitochondria inhibits ATP synthesis . This disruption of energy production pathways leads to cellular energy depletion .
Pharmacokinetics
It’s known that this compound is used primarily as a topical antibiotic , suggesting that its bioavailability may be localized and dependent on the application site.
Result of Action
The action of this compound leads to significant molecular and cellular effects. The disruption of the ion concentration gradients and the inhibition of ATP synthesis lead to cellular energy depletion . This energy depletion can induce G1 phase accumulation , leading to cell growth inhibition . In addition, the action of this compound is associated with the formation of hydroxyl radicals , which can cause further damage to the bacterial cell.
Action Environment
Like other antimicrobial peptides, the activity of this compound may be influenced by factors such as ph, temperature, and the presence of other ions or molecules
Biochemical Analysis
Biochemical Properties
Gramicidin plays a crucial role in biochemical reactions by forming transmembrane channels that allow the passage of monovalent cations such as sodium and potassium. This disrupts the ion gradient across the cell membrane, leading to cell death. This compound interacts with various biomolecules, including lipid bilayers and membrane proteins. The interaction with lipid bilayers is particularly significant as it facilitates the formation of ion channels, which is essential for its antimicrobial activity .
Cellular Effects
This compound exerts profound effects on various types of cells and cellular processes. It influences cell function by disrupting the ion gradients across the plasma membrane, leading to the loss of intracellular solutes such as potassium and amino acids. This disruption affects cell signaling pathways, gene expression, and cellular metabolism. This compound has been shown to inhibit oxidative phosphorylation and glycolysis, leading to a reduction in ATP levels and cellular energy depletion .
Molecular Mechanism
The molecular mechanism of this compound involves its insertion into bacterial membranes, where it forms transmembrane channels. These channels disrupt the membrane potential and permeability, leading to cell death. This compound binds to and inserts itself into the lipid bilayer, forming a β-helix structure that spans the membrane. This structure allows the passage of monovalent cations, disrupting the ion gradient and leading to cellular dysfunction .
Temporal Effects in Laboratory Settings
In laboratory settings, the effects of this compound change over time. This compound is relatively stable, but its activity can be influenced by factors such as temperature and pH. Over time, this compound can degrade, leading to a reduction in its antimicrobial efficacy. Long-term studies have shown that this compound can cause persistent changes in cellular function, including alterations in membrane potential and ion gradients .
Dosage Effects in Animal Models
The effects of this compound vary with different dosages in animal models. At low doses, this compound can effectively inhibit bacterial growth without causing significant toxicity. At high doses, this compound can cause adverse effects such as hemolysis and toxicity to host cells. Threshold effects have been observed, where a certain dosage is required to achieve antimicrobial activity without causing harm to the host .
Metabolic Pathways
This compound is involved in metabolic pathways related to ion transport and cellular energy production. It interacts with enzymes and cofactors involved in oxidative phosphorylation and glycolysis. By disrupting the ion gradients, this compound affects the metabolic flux and levels of metabolites such as ATP. This disruption can lead to cellular energy depletion and metabolic dysfunction .
Transport and Distribution
This compound is transported and distributed within cells and tissues through its interaction with lipid bilayers. It is lipophilic and can easily pass through cell membranes. This compound forms homo-dimers that function as ion channels, facilitating its transport across membranes. This distribution is crucial for its antimicrobial activity, as it allows this compound to reach and disrupt bacterial membranes .
Subcellular Localization
This compound localizes to specific subcellular compartments, particularly the plasma membrane and mitochondria. In the plasma membrane, this compound forms ion channels that disrupt ion gradients. In mitochondria, this compound can depolarize the inner mitochondrial membrane, leading to inhibition of ATP synthesis and induction of mitophagy. These localization patterns are essential for its function as an antimicrobial agent .
Preparation Methods
Gramicidin D is produced by the fermentation of Bacillus brevis. The fermentation broth is then subjected to extraction and purification processes to isolate the antibiotic compounds. The synthetic routes involve the use of solid-phase peptide synthesis techniques to assemble the 15-residue peptides with alternating D and L amino acids .
Chemical Reactions Analysis
Gramicidin D undergoes various chemical reactions, including oxidation, reduction, and substitution. Common reagents used in these reactions include strong oxidizing agents, reducing agents, and nucleophiles. The major products formed from these reactions depend on the specific conditions and reagents used. For example, oxidation of this compound D can lead to the formation of oxidized peptide derivatives .
Scientific Research Applications
Gramicidin D has a wide range of scientific research applications. In chemistry, it is used as a model system for studying ion conduction and protein-lipid interactions. In biology, it is used to study the structure and function of ion channels. In medicine, this compound D is used as a topical antibiotic for treating skin lesions, surface wounds, and eye infections. It has also been studied for its potential use in combating antibiotic resistance and inhibiting SARS-CoV-2 PLpro .
Comparison with Similar Compounds
Gramicidin D is unique due to its composition of three different antibiotic compounds and its ability to form helical pores in bacterial membranes. Similar compounds include this compound S, which is a cyclic peptide with antimicrobial activity, and other antimicrobial peptides such as polymyxin B and neomycin. These compounds also target bacterial membranes but differ in their structure and mechanism of action .
Biological Activity
Gramicidin is a peptide antibiotic first isolated from Bacillus brevis that exhibits significant biological activity, particularly against Gram-positive bacteria. Its primary mechanism of action involves the formation of ion channels in cellular membranes, leading to disruption of ion gradients and ultimately cell death. This article explores the biological activities of this compound, focusing on its antimicrobial properties, cytotoxic effects on cancer cells, and potential therapeutic applications.
1. Antimicrobial Properties
This compound is renowned for its potent antimicrobial activity , especially against Gram-positive bacteria such as Staphylococcus aureus and Bacillus subtilis. The mechanism of action primarily involves the formation of ion channels that disrupt membrane integrity.
Table 1: Antimicrobial Activity of this compound A
Bacterial Strain | Minimum Inhibitory Concentration (MIC) | Mechanism of Action |
---|---|---|
Staphylococcus aureus | 0.5 μg/mL | Ion channel formation |
Bacillus subtilis | 1.0 μg/mL | Membrane permeabilization |
Listeria monocytogenes | 0.25 μg/mL | Disruption of ion gradients |
Recent studies have indicated that this compound A (gA) generates hydroxyl radicals during bacterial treatment, suggesting an additional mechanism contributing to its bactericidal effects beyond mere membrane disruption .
2. Cytotoxic Effects on Cancer Cells
Emerging research has highlighted the anticancer properties of this compound, particularly its ability to inhibit the proliferation of various cancer cell lines. A recent study demonstrated that this compound significantly reduces the growth of ovarian cancer cells through apoptosis induction.
Case Study: this compound's Effect on Ovarian Cancer Cells
- Cell Lines Studied : OVCAR8, SKOV3, A2780
- Methods : WST-1 assay, TUNEL assay, DNA agarose gel electrophoresis, flow cytometry, Western blot analysis.
- Findings :
3. Structure-Activity Relationship Studies
Research has focused on modifying this compound to enhance its therapeutic index while reducing cytotoxicity. High-throughput screening has led to the identification of several analogues with altered biological activity profiles.
Table 2: this compound Analogues and Their Biological Activities
Analogue Name | Cytotoxicity (IC50) | Antibacterial Activity | Hemolytic Activity |
---|---|---|---|
Analogue 1 | 0.05 μM | Effective | Low |
Analogue 2 | 0.10 μM | Moderate | Moderate |
Analogue 3 | >1 μM | Ineffective | High |
These studies demonstrate the potential for developing this compound derivatives that maintain antimicrobial efficacy while minimizing adverse effects on mammalian cells .
Properties
Key on ui mechanism of action |
Gramicidin D binds to and inserts itself into bacterial membranes (with a strong preference to gram-positive cell membranes). This results in membrane disruption and permeabilization (it acts as a channel). This leads to (i) loss of intracellular solutes (e.g., K+ and amino acids); (ii) dissipation of the transmembrane potential; (iii) inhibition of respiration; (iv) a reduction in ATP pools; and (v) inhibition of DNA, RNA, and protein synthesis, which leads to cell death. |
---|---|
CAS No. |
1393-88-0 |
Molecular Formula |
C96H135N19O16 |
Molecular Weight |
1811.2 g/mol |
IUPAC Name |
(2R)-2-[[2-[[(2S)-2-formamido-3-methylbutanoyl]amino]acetyl]amino]-N-[(2S)-1-[[(2R)-1-[[(2S)-1-[[(2R)-1-[[(2S)-1-[[(2R)-1-[[(2S)-1-[[(2R)-1-[[(2S)-1-[[(2R)-1-[[(2S)-1-(2-hydroxyethylamino)-3-(1H-indol-3-yl)-1-oxopropan-2-yl]amino]-4-methyl-1-oxopentan-2-yl]amino]-3-(1H-indol-3-yl)-1-oxopropan-2-yl]amino]-4-methyl-1-oxopentan-2-yl]amino]-3-(1H-indol-3-yl)-1-oxopropan-2-yl]amino]-4-methyl-1-oxopentan-2-yl]amino]-3-(1H-indol-3-yl)-1-oxopropan-2-yl]amino]-3-methyl-1-oxobutan-2-yl]amino]-3-methyl-1-oxobutan-2-yl]amino]-3-methyl-1-oxobutan-2-yl]amino]-1-oxopropan-2-yl]-4-methylpentanamide |
InChI |
InChI=1S/C96H135N19O16/c1-50(2)36-71(105-79(118)48-102-93(128)80(54(9)10)103-49-117)86(121)104-58(17)84(119)113-82(56(13)14)95(130)115-83(57(15)16)96(131)114-81(55(11)12)94(129)112-78(43-62-47-101-70-33-25-21-29-66(62)70)92(127)108-74(39-53(7)8)89(124)111-77(42-61-46-100-69-32-24-20-28-65(61)69)91(126)107-73(38-52(5)6)88(123)110-76(41-60-45-99-68-31-23-19-27-64(60)68)90(125)106-72(37-51(3)4)87(122)109-75(85(120)97-34-35-116)40-59-44-98-67-30-22-18-26-63(59)67/h18-33,44-47,49-58,71-78,80-83,98-101,116H,34-43,48H2,1-17H3,(H,97,120)(H,102,128)(H,103,117)(H,104,121)(H,105,118)(H,106,125)(H,107,126)(H,108,127)(H,109,122)(H,110,123)(H,111,124)(H,112,129)(H,113,119)(H,114,131)(H,115,130)/t58-,71+,72+,73+,74+,75-,76-,77-,78-,80-,81+,82+,83-/m0/s1 |
InChI Key |
NDAYQJDHGXTBJL-MWWSRJDJSA-N |
Isomeric SMILES |
C[C@@H](C(=O)N[C@H](C(C)C)C(=O)N[C@@H](C(C)C)C(=O)N[C@H](C(C)C)C(=O)N[C@@H](CC1=CNC2=CC=CC=C21)C(=O)N[C@H](CC(C)C)C(=O)N[C@@H](CC3=CNC4=CC=CC=C43)C(=O)N[C@H](CC(C)C)C(=O)N[C@@H](CC5=CNC6=CC=CC=C65)C(=O)N[C@H](CC(C)C)C(=O)N[C@@H](CC7=CNC8=CC=CC=C87)C(=O)NCCO)NC(=O)[C@@H](CC(C)C)NC(=O)CNC(=O)[C@H](C(C)C)NC=O |
Canonical SMILES |
CC(C)CC(C(=O)NC(C)C(=O)NC(C(C)C)C(=O)NC(C(C)C)C(=O)NC(C(C)C)C(=O)NC(CC1=CNC2=CC=CC=C21)C(=O)NC(CC(C)C)C(=O)NC(CC3=CNC4=CC=CC=C43)C(=O)NC(CC(C)C)C(=O)NC(CC5=CNC6=CC=CC=C65)C(=O)NC(CC(C)C)C(=O)NC(CC7=CNC8=CC=CC=C87)C(=O)NCCO)NC(=O)CNC(=O)C(C(C)C)NC=O |
Origin of Product |
United States |
Disclaimer and Information on In-Vitro Research Products
Please be aware that all articles and product information presented on BenchChem are intended solely for informational purposes. The products available for purchase on BenchChem are specifically designed for in-vitro studies, which are conducted outside of living organisms. In-vitro studies, derived from the Latin term "in glass," involve experiments performed in controlled laboratory settings using cells or tissues. It is important to note that these products are not categorized as medicines or drugs, and they have not received approval from the FDA for the prevention, treatment, or cure of any medical condition, ailment, or disease. We must emphasize that any form of bodily introduction of these products into humans or animals is strictly prohibited by law. It is essential to adhere to these guidelines to ensure compliance with legal and ethical standards in research and experimentation.