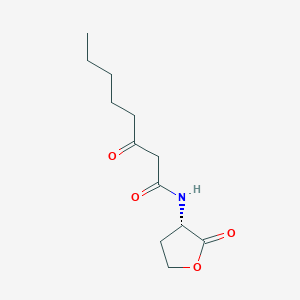
N-(3-Oxooctanoyl)-L-homoserine lactone
Overview
Description
N-(3-Oxooctanoyl)-L-homoserine lactone (3OC8-HSL) is a quorum sensing (QS) signal molecule belonging to the N-acyl homoserine lactone (AHL) family. It features an 8-carbon acyl chain with a 3-oxo modification and a homoserine lactone ring. With a molecular formula of C₁₂H₁₉NO₄ and a molecular weight of 241.287 g/mol, it is widely studied for its role in bacterial communication, biofilm regulation, and interspecies interactions .
3OC8-HSL is synthesized by bacteria such as Pseudomonas aeruginosa and regulates virulence factors, biofilm formation, and extracellular polymeric substance (EPS) production. Its structural stability (≥97% purity) and solubility in chloroform (50 mg/mL) make it a standard in QS research .
Preparation Methods
Synthetic Routes and Reaction Conditions
Chemical Reactions Analysis
Hydrolysis of the Lactone Ring
The lactone ring in 3OC8-HSL is susceptible to hydrolysis under physiological or aqueous conditions, yielding a carboxylic acid derivative. This reaction is pH-dependent and occurs spontaneously in aqueous environments or through enzymatic catalysis.
Amide Bond Cleavage
The amide bond linking the homoserine lactone and 3-oxooctanoyl moieties can hydrolyze, producing two fragments: L-homoserine lactone and 3-oxooctanoic acid.
Redox Reactions
While direct experimental data on oxidation/reduction of 3OC8-HSL is limited in the reviewed sources, structural analogs suggest potential reactivity:
-
Oxidation : The 3-oxo group may undergo further oxidation to form carboxylates under strong oxidizing conditions (e.g., KMnO₄).
-
Reduction : The ketone group could be reduced to a hydroxyl group using agents like NaBH₄, forming N-(3-hydroxyoctanoyl)-L-homoserine lactone.
Comparative Reactivity of AHL Derivatives
The reactivity of 3OC8-HSL differs from related acyl-homoserine lactones (AHLs) due to its acyl chain length and functional groups:
Mechanistic Insights
-
Lactone Hydrolysis : Proceeds via nucleophilic attack by water at the ester carbonyl, facilitated by alkaline conditions or enzymes.
-
Amide Cleavage : Requires hydrolytic enzymes (e.g., acylase I) or prolonged exposure to basic conditions.
Biological Implications of Hydrolysis
Hydrolysis products retain signaling capabilities in plants:
-
3-Oxooctanoic acid influences auxin-like responses, promoting adventitious root formation .
-
L-Homoserine derivatives trigger H₂O₂ and NO synthesis in plant tissues, mimicking stress responses .
Synthetic Modifications
Though not directly observed in the reviewed studies, proposed modifications include:
-
Acyl Chain Substitution : Replacing the octanoyl group with fluorinated or branched chains to alter receptor binding.
-
Lactone Stabilization : Methylation of the lactone oxygen to reduce hydrolysis rates.
Scientific Research Applications
Quorum Sensing in Bacteria
Role as a Quorum Sensing Molecule
- 3OC8-HSL functions as an autoinducer in bacterial communication, regulating gene expression related to virulence and biofilm formation. It is particularly significant in Pseudomonas aeruginosa, where it modulates the expression of virulence factors and biofilm development .
Case Study: Biofilm Formation
- Research indicates that 3OC8-HSL enhances biofilm formation in Pseudomonas aeruginosa. The compound's presence leads to the upregulation of genes involved in extracellular polymeric substance (EPS) production, which is essential for biofilm integrity .
Plant-Microbe Interactions
Induction of Plant Defense Mechanisms
- 3OC8-HSL has been shown to prime plant defenses against pathogens. For instance, it enhances resistance in Arabidopsis thaliana against Pseudomonas syringae through the salicylic acid pathway, demonstrating its role in plant immunity .
Case Study: Resistance Priming
- A study revealed that treatment with 3OC8-HSL resulted in differential expression of proteins involved in flavonoid synthesis and oxidative stress response in Medicago truncatula, indicating its potential as a biopesticide or plant growth enhancer .
Cancer Research
Cytotoxic Effects on Cancer Cells
- 3OC8-HSL exhibits cytotoxic properties against various cancer cell lines. It has been observed to induce apoptosis in pancreatic carcinoma cells, reducing cell viability and inhibiting migration .
Case Study: Breast Cancer Microenvironment
- A study explored the interactions between 3OC8-HSL and breast tumor microenvironments, revealing that this compound influences cancer cell viability and proliferation under hypoxic conditions. This highlights its potential role in modulating tumor behavior through microbial interactions .
Immunomodulatory Effects
Impact on Immune Cells
- Research indicates that 3OC8-HSL can modulate immune responses by inducing apoptosis in macrophages and neutrophils. This property may have implications for understanding bacterial pathogenesis and developing immunotherapeutics .
Case Study: Apoptosis Induction
- In vitro studies demonstrated that exposure to varying concentrations of 3OC8-HSL led to significant apoptosis in immune cells, suggesting its dual role as a signaling molecule and a potential therapeutic agent .
Data Tables
Mechanism of Action
N-3-oxo-octanoyl-L-Homoserine lactone functions as an autoinducer in quorum sensing. It binds to specific receptor proteins in bacteria, leading to the activation of gene expression related to various physiological processes, including virulence, biofilm formation, and antibiotic resistance . The molecular targets include LuxR-type receptors, which regulate the transcription of target genes .
Comparison with Similar Compounds
Structural Comparison with Similar AHLs
AHLs vary in acyl chain length (C4–C16) and substitutions (e.g., 3-oxo, 3-hydroxy). Below is a structural comparison of 3OC8-HSL with key analogs:
Key Observations :
- The 3-oxo group enhances receptor binding affinity (e.g., LuxR-type proteins) compared to unmodified AHLs .
- Longer acyl chains (e.g., 3OC12-HSL) increase hydrophobicity, influencing membrane interactions and host cell effects .
Role in Bacterial Communication
- 3OC8-HSL: Critical for P. aeruginosa biofilm maturation and EPS production. It acts as a self-inducer and antagonist in dual-species biofilms .
- 3OC6-HSL : Regulates carbapenem antibiotic synthesis in Erwinia carotovora via cross-feeding between bacterial mutants .
- 3OC12-HSL : Induces apoptosis in mammalian cells (e.g., endothelial cells, mast cells) via mitochondrial pathways and RIPK1 activation .
Host Organism Responses
- Plants : Arabidopsis thaliana exhibits differential root growth responses to 3OC8-HSL (neutral) versus 3OC12-HSL (inhibitory) .
Enzymatic Degradation and Stability
AHL-lactonases and acylases degrade AHLs, disrupting QS. Below is a kinetic comparison:
Key Findings :
- AiiA from B. subtilis shows high catalytic efficiency and thermostability against 3OC8-HSL, making it a candidate for QS quenching .
- 3OC12-HSL is more resistant to enzymatic degradation due to its longer acyl chain, prolonging its biological activity .
Contrasting Effects in Different Species
- Biofilm Formation: 3OC8-HSL and C8-HSL (unmodified) both enhance biofilm formation in Pseudomonas and Microcystis, but 3OC8-HSL specifically strengthens colony formation in cyanobacteria .
Biological Activity
N-(3-Oxooctanoyl)-L-homoserine lactone (3OC8-HSL) is a significant quorum-sensing (QS) signaling molecule produced by various Gram-negative bacteria, particularly Pseudomonas aeruginosa. This compound plays a crucial role in intercellular communication, influencing a range of biological activities in both bacterial and eukaryotic cells. This article provides a comprehensive overview of the biological activities associated with 3OC8-HSL, including its effects on cellular processes, immune responses, and potential therapeutic applications.
Quorum Sensing and Bacterial Communication
Quorum sensing is a process through which bacteria communicate and coordinate their behavior based on population density. 3OC8-HSL is one of the acyl-homoserine lactones (AHLs) that facilitate this communication. It is involved in regulating various physiological functions, including biofilm formation, virulence factor production, and bioluminescence in bacteria.
Key Functions of 3OC8-HSL:
- Biofilm Formation : 3OC8-HSL promotes the synthesis of extracellular polymeric substances (EPS) in bacterial biofilms, enhancing their stability and resilience against environmental stressors .
- Virulence Factor Regulation : This AHL modulates the expression of genes responsible for virulence factors, thereby influencing pathogenicity in host organisms .
Effects on Eukaryotic Cells
Recent studies have revealed that 3OC8-HSL also interacts with eukaryotic cells, leading to various biological responses. The following sections detail these interactions and their implications.
Immunomodulatory Effects
3OC8-HSL exhibits significant immunomodulatory properties. It has been shown to influence the behavior of immune cells such as lymphocytes and macrophages:
- Lymphocyte Proliferation : At varying concentrations, 3OC8-HSL can inhibit or promote lymphocyte proliferation. High concentrations tend to suppress proliferation and cytokine production (e.g., TNF-α and IL-12), while low concentrations may enhance antibody production .
- Macrophage Activation : In macrophages, 3OC8-HSL can modulate inflammatory responses. It has been observed to decrease pro-inflammatory cytokines like TNF-α while promoting anti-inflammatory cytokines such as IL-10 . This duality suggests a complex role in managing inflammation and immune responses.
Apoptosis Induction
Studies indicate that 3OC8-HSL can induce apoptosis in certain cell types. For instance, it triggers mitochondrial outer membrane permeabilization, leading to the activation of intrinsic apoptotic pathways in mouse embryonic fibroblasts . This mechanism highlights its potential as a therapeutic agent in cancer research, where modulation of cell death pathways is crucial.
Case Studies and Research Findings
Several studies have investigated the biological activity of 3OC8-HSL across different contexts:
- Breast Cancer Microenvironment : Research demonstrated that quorum-sensing molecules like 3OC8-HSL could interact with breast cancer microenvironments, influencing tumor progression through modulation of local immune responses .
- Plant Responses : In plant systems, 3OC8-HSL has been shown to prime resistance against pathogens by enhancing root development and activating specific signaling pathways involving nitric oxide (NO) and cyclic GMP (cGMP) .
Comparative Biological Activity Table
Q & A
Basic Research Questions
Q. What methods are recommended for detecting and quantifying 3-oxo-C8-HSL in bacterial cultures?
Answer:
- Thin-Layer Chromatography (TLC): Separate 3-oxo-C8-HSL using silica gel TLC plates with a chloroform:methanol solvent system. Use Agrobacterium tumefaciens reporter strains (e.g., lacZ fusion) to visualize spots via β-galactosidase activity .
- Quantification: Calibrate with synthetic 3-oxo-C8-HSL standards. Spot intensity correlates with concentration, validated via densitometry .
- Confirmatory Techniques: Use HPLC coupled with mass spectrometry (HPLC-MS) for structural confirmation. Electrospray ionization (ESI) in positive ion mode provides accurate mass-to-charge ratios (e.g., m/z 242.2 for [M+H]⁺) .
Q. How does 3-oxo-C8-HSL influence bacterial biofilm formation?
Answer:
- Experimental Design: Treat Pseudomonas aeruginosa cultures with 3-oxo-C8-HSL (0.1–10 µM) under varying pH (5.0–8.0), salinity (0–5% NaCl), and temperature (25–37°C). Assess biofilm biomass via crystal violet staining .
- EPS Analysis: Quantify extracellular polymeric substances (EPS) using phenol-sulfuric acid (carbohydrates) and Bradford assays (proteins). 3-oxo-C8-HSL enhances EPS production at neutral pH and 30°C .
Q. What are the best practices for synthesizing and handling 3-oxo-C8-HSL in the lab?
Answer:
- Synthesis: Use L-homoserine lactone and 3-oxooctanoyl chloride in anhydrous dichloromethane with coupling agents (e.g., DCC/DMAP). Purify via silica gel chromatography (ethyl acetate:hexane, 3:7) .
- Handling: Store at −20°C in chloroform (50 mg/mL). Avoid aqueous buffers to prevent hydrolysis. Use glass vials to minimize adsorption .
Advanced Research Questions
Q. How can researchers resolve contradictory data on 3-oxo-C8-HSL bioactivity across studies?
Answer:
- Degradation Analysis: Screen for AHL-modifying enzymes (e.g., Rhodococcus erythropolis oxidoreductases) in bacterial co-cultures. Monitor 3-oxo-C8-HSL conversion to 3-hydroxy-C8-HSL via LC-MS .
- Reporter Strain Specificity: Validate A. tumefaciens reporter sensitivity to 3-oxo-C8-HSL vs. analogs. Use competitive binding assays with LuxR-type receptors .
Q. What strategies are effective in studying 3-oxo-C8-HSL interactions with non-canonical bacterial receptors?
Answer:
- Genetic Knockouts: Construct luxR homolog mutants (e.g., lasR in P. aeruginosa). Test 3-oxo-C8-HSL-induced gene expression via RNA-seq or GFP reporters .
- Surface Plasmon Resonance (SPR): Immobilize recombinant receptors on sensor chips. Measure binding kinetics (KD) with 3-oxo-C8-HSL at 0.1–100 µM .
Q. How do environmental factors modulate 3-oxo-C8-HSL stability and signaling efficiency?
Answer:
- Stability Assays: Incubate 3-oxo-C8-HSL in soil/water microcosms (pH 4–9, 4–37°C). Quantify degradation via LC-MS. Half-life decreases >50% at pH >8.0 .
- Signal Potency: Use microfluidics to simulate dynamic environments (e.g., nutrient gradients). Couple with micro-scale bioreporters for real-time quorum sensing readouts .
Q. What enzymatic pathways degrade 3-oxo-C8-HSL, and how can they be characterized?
Answer:
- Enzyme Screening: Isolate AHL acylases (e.g., aiiD from Ralstonia sp.) via activity-guided fractionation. Test substrate specificity using 3-oxo-C8-HSL and analogs (C6–C12) .
- Kinetic Studies: Monitor hydrolysis via HPLC (homoserine lactone release) and NADH-coupled assays for oxidoreductases (e.g., 340 nm absorbance) .
Q. Methodological Notes
- Data Validation: Always cross-verify TLC/HPLC results with reporter assays to confirm biological activity .
- Storage: Aliquot 3-oxo-C8-HSL to avoid freeze-thaw cycles. Use inert gas (N2) to prevent oxidation in solution .
- Controls: Include synthetic 3-oxo-C8-HSL (≥97% purity) and lasI mutant strains as negative controls in biofilm studies .
Properties
IUPAC Name |
3-oxo-N-[(3S)-2-oxooxolan-3-yl]octanamide | |
---|---|---|
Source | PubChem | |
URL | https://pubchem.ncbi.nlm.nih.gov | |
Description | Data deposited in or computed by PubChem | |
InChI |
InChI=1S/C12H19NO4/c1-2-3-4-5-9(14)8-11(15)13-10-6-7-17-12(10)16/h10H,2-8H2,1H3,(H,13,15)/t10-/m0/s1 | |
Source | PubChem | |
URL | https://pubchem.ncbi.nlm.nih.gov | |
Description | Data deposited in or computed by PubChem | |
InChI Key |
FXCMGCFNLNFLSH-JTQLQIEISA-N | |
Source | PubChem | |
URL | https://pubchem.ncbi.nlm.nih.gov | |
Description | Data deposited in or computed by PubChem | |
Canonical SMILES |
CCCCCC(=O)CC(=O)NC1CCOC1=O | |
Source | PubChem | |
URL | https://pubchem.ncbi.nlm.nih.gov | |
Description | Data deposited in or computed by PubChem | |
Isomeric SMILES |
CCCCCC(=O)CC(=O)N[C@H]1CCOC1=O | |
Source | PubChem | |
URL | https://pubchem.ncbi.nlm.nih.gov | |
Description | Data deposited in or computed by PubChem | |
Molecular Formula |
C12H19NO4 | |
Source | PubChem | |
URL | https://pubchem.ncbi.nlm.nih.gov | |
Description | Data deposited in or computed by PubChem | |
DSSTOX Substance ID |
DTXSID60163802 | |
Record name | N-(beta-Oxooctan-1-oyl)homoserine lactone | |
Source | EPA DSSTox | |
URL | https://comptox.epa.gov/dashboard/DTXSID60163802 | |
Description | DSSTox provides a high quality public chemistry resource for supporting improved predictive toxicology. | |
Molecular Weight |
241.28 g/mol | |
Source | PubChem | |
URL | https://pubchem.ncbi.nlm.nih.gov | |
Description | Data deposited in or computed by PubChem | |
CAS No. |
147795-39-9 | |
Record name | N-(beta-Oxooctan-1-oyl)homoserine lactone | |
Source | ChemIDplus | |
URL | https://pubchem.ncbi.nlm.nih.gov/substance/?source=chemidplus&sourceid=0147795399 | |
Description | ChemIDplus is a free, web search system that provides access to the structure and nomenclature authority files used for the identification of chemical substances cited in National Library of Medicine (NLM) databases, including the TOXNET system. | |
Record name | N-(beta-Oxooctan-1-oyl)homoserine lactone | |
Source | EPA DSSTox | |
URL | https://comptox.epa.gov/dashboard/DTXSID60163802 | |
Description | DSSTox provides a high quality public chemistry resource for supporting improved predictive toxicology. | |
Retrosynthesis Analysis
AI-Powered Synthesis Planning: Our tool employs the Template_relevance Pistachio, Template_relevance Bkms_metabolic, Template_relevance Pistachio_ringbreaker, Template_relevance Reaxys, Template_relevance Reaxys_biocatalysis model, leveraging a vast database of chemical reactions to predict feasible synthetic routes.
One-Step Synthesis Focus: Specifically designed for one-step synthesis, it provides concise and direct routes for your target compounds, streamlining the synthesis process.
Accurate Predictions: Utilizing the extensive PISTACHIO, BKMS_METABOLIC, PISTACHIO_RINGBREAKER, REAXYS, REAXYS_BIOCATALYSIS database, our tool offers high-accuracy predictions, reflecting the latest in chemical research and data.
Strategy Settings
Precursor scoring | Relevance Heuristic |
---|---|
Min. plausibility | 0.01 |
Model | Template_relevance |
Template Set | Pistachio/Bkms_metabolic/Pistachio_ringbreaker/Reaxys/Reaxys_biocatalysis |
Top-N result to add to graph | 6 |
Feasible Synthetic Routes
Disclaimer and Information on In-Vitro Research Products
Please be aware that all articles and product information presented on BenchChem are intended solely for informational purposes. The products available for purchase on BenchChem are specifically designed for in-vitro studies, which are conducted outside of living organisms. In-vitro studies, derived from the Latin term "in glass," involve experiments performed in controlled laboratory settings using cells or tissues. It is important to note that these products are not categorized as medicines or drugs, and they have not received approval from the FDA for the prevention, treatment, or cure of any medical condition, ailment, or disease. We must emphasize that any form of bodily introduction of these products into humans or animals is strictly prohibited by law. It is essential to adhere to these guidelines to ensure compliance with legal and ethical standards in research and experimentation.