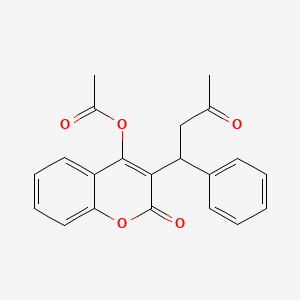
Warfarin acetate
- Click on QUICK INQUIRY to receive a quote from our team of experts.
- With the quality product at a COMPETITIVE price, you can focus more on your research.
Overview
Description
Warfarin acetate is a widely used anticoagulant that inhibits blood clot formation. It is commonly prescribed under various brand names, including Coumadin and Jantoven . This compound functions by inhibiting the synthesis of vitamin K-dependent clotting factors, making it an essential medication for preventing thromboembolic events such as deep vein thrombosis, pulmonary embolism, and stroke .
Preparation Methods
Synthetic Routes and Reaction Conditions: Warfarin acetate is synthesized through a multi-step process. The primary synthetic route involves the condensation of 4-hydroxycoumarin with benzylideneacetone under basic conditions. This reaction forms the intermediate compound, which is then acetylated to produce this compound .
Industrial Production Methods: Industrial production of this compound typically involves large-scale batch processes. The reaction conditions are carefully controlled to ensure high yield and purity. The final product is subjected to rigorous quality control measures, including chromatographic analysis, to confirm its chemical composition and potency .
Chemical Reactions Analysis
Types of Reactions: Warfarin acetate undergoes various chemical reactions, including hydroxylation, reduction, and substitution. These reactions are primarily catalyzed by cytochrome P450 enzymes in the liver .
Common Reagents and Conditions:
Hydroxylation: Catalyzed by cytochrome P450 enzymes, particularly CYP2C9 and CYP3A4, under physiological conditions.
Reduction: Involves the use of reducing agents such as sodium borohydride.
Substitution: Typically occurs in the presence of strong nucleophiles under basic conditions.
Major Products: The major products formed from these reactions include various hydroxylated metabolites, such as 7-hydroxywarfarin and 10-hydroxywarfarin .
Scientific Research Applications
Clinical Applications
-
Prevention of Thromboembolic Events
- Warfarin is primarily used to prevent venous thromboembolism (VTE) in patients undergoing orthopedic surgeries such as hip or knee replacements.
- It is also indicated for patients with non-valvular atrial fibrillation to reduce the risk of stroke.
-
Management of Existing Thromboembolic Conditions
- It is used in the treatment of deep vein thrombosis (DVT) and pulmonary embolism (PE).
- Patients with mechanical heart valves are often prescribed warfarin to prevent thrombus formation.
-
Long-term Anticoagulation Therapy
- Warfarin is utilized for long-term anticoagulation in patients with recurrent thromboembolic events or those with specific genetic predispositions to clotting disorders.
Pharmacokinetics and Drug Interactions
Warfarin exhibits significant variability in pharmacokinetics among individuals due to genetic factors, diet, and concomitant medications. The S-enantiomer of warfarin is approximately five times more potent than the R-enantiomer. Various studies have highlighted interactions with drugs such as eslicarbazepine acetate and norethindrone, which can alter warfarin's effectiveness and safety profile .
Table 1: Key Pharmacokinetic Parameters
Parameter | Value |
---|---|
Half-life | 36-42 hours |
Bioavailability | 100% (oral administration) |
Protein binding | 97% |
Metabolism | Hepatic (CYP2C9) |
Case Studies
- Case Study on Warfarin Resistance
- Drug Interaction Case Study
Adverse Effects and Monitoring
The primary adverse effect associated with warfarin therapy is bleeding, which necessitates regular monitoring of INR levels. Other potential complications include skin necrosis and osteoporosis due to long-term use .
Table 2: Common Adverse Effects
Adverse Effect | Incidence |
---|---|
Major bleeding | 1-3% per year |
Skin necrosis | Rare |
Osteoporosis | Long-term use |
Mechanism of Action
Warfarin acetate exerts its anticoagulant effects by inhibiting the enzyme vitamin K epoxide reductase. This inhibition prevents the regeneration of active vitamin K1, which is essential for the synthesis of clotting factors II, VII, IX, and X. As a result, the blood’s ability to clot is significantly reduced .
Comparison with Similar Compounds
Dicoumarol: Another anticoagulant that also inhibits vitamin K-dependent clotting factors.
Acenocoumarol: Similar in structure and function to warfarin acetate but with a shorter half-life.
Phenprocoumon: A long-acting anticoagulant with a similar mechanism of action
Uniqueness of this compound: this compound is unique due to its well-established pharmacokinetic profile, extensive clinical use, and the availability of a reversal agent (vitamin K1). Its ability to be administered orally and its relatively predictable dose-response relationship make it a preferred choice for long-term anticoagulation therapy .
Biological Activity
Warfarin acetate, a derivative of warfarin, is widely recognized for its anticoagulant properties. It functions primarily by inhibiting the vitamin K epoxide reductase complex (VKORC1), which is essential for the synthesis of vitamin K-dependent clotting factors. This article delves into the biological activity of this compound, examining its mechanisms, pharmacokinetics, and case studies to illustrate its clinical implications.
This compound exerts its anticoagulant effects through several key mechanisms:
- Inhibition of VKORC1 : Warfarin binds to VKORC1, preventing the conversion of vitamin K epoxide back to its active form. This inhibition reduces the synthesis of active clotting factors II (prothrombin), VII, IX, and X, which are crucial for normal blood coagulation .
- Impact on Coagulation Factors : The anticoagulant effect is not immediate; it depends on the half-lives of the coagulation factors. Factors C and S degrade first due to their shorter half-lives (8 and 24 hours, respectively), leading to a transient hypercoagulable state until new factors are synthesized .
- Pharmacogenetics : Variants in genes such as CYP2C9 and VKORC1 significantly influence warfarin metabolism and dosing requirements. For instance, certain polymorphisms in these genes can lead to reduced enzyme activity, necessitating lower doses of warfarin for effective anticoagulation .
Pharmacokinetics
This compound is characterized by its narrow therapeutic index and significant inter-individual variability in response. Key pharmacokinetic properties include:
- Absorption : Warfarin is rapidly absorbed from the gastrointestinal tract.
- Distribution : It is highly protein-bound (approximately 99%), primarily to albumin.
- Metabolism : The drug undergoes hepatic metabolism via cytochrome P450 enzymes (mainly CYP2C9 for the S-enantiomer) into inactive metabolites .
- Elimination : The elimination half-life ranges from 20 to 60 hours, depending on individual metabolic rates.
Case Studies
Several clinical case studies highlight the complexities and challenges associated with warfarin therapy:
- Warfarin Resistance :
- Pharmacogenetic Testing :
- Interactions with Other Medications :
Research Findings
Recent studies have further elucidated the biological activity of this compound:
- Esterase Activity Modulation : A study indicated that warfarin influences albumin's esterase activity, which may have implications for drug metabolism and interaction profiles in vivo .
- Comparative Efficacy : A study comparing warfarin with rivaroxaban in patients with atrial fibrillation found no significant differences in embolic events or bleeding complications over a follow-up period, suggesting that while both drugs are effective, patient-specific factors must guide therapy choice .
Properties
CAS No. |
5979-00-0 |
---|---|
Molecular Formula |
C21H18O5 |
Molecular Weight |
350.4 g/mol |
IUPAC Name |
[2-oxo-3-(3-oxo-1-phenylbutyl)chromen-4-yl] acetate |
InChI |
InChI=1S/C21H18O5/c1-13(22)12-17(15-8-4-3-5-9-15)19-20(25-14(2)23)16-10-6-7-11-18(16)26-21(19)24/h3-11,17H,12H2,1-2H3 |
InChI Key |
AZJMOTKZYSVMRV-UHFFFAOYSA-N |
Canonical SMILES |
CC(=O)CC(C1=CC=CC=C1)C2=C(C3=CC=CC=C3OC2=O)OC(=O)C |
Origin of Product |
United States |
Disclaimer and Information on In-Vitro Research Products
Please be aware that all articles and product information presented on BenchChem are intended solely for informational purposes. The products available for purchase on BenchChem are specifically designed for in-vitro studies, which are conducted outside of living organisms. In-vitro studies, derived from the Latin term "in glass," involve experiments performed in controlled laboratory settings using cells or tissues. It is important to note that these products are not categorized as medicines or drugs, and they have not received approval from the FDA for the prevention, treatment, or cure of any medical condition, ailment, or disease. We must emphasize that any form of bodily introduction of these products into humans or animals is strictly prohibited by law. It is essential to adhere to these guidelines to ensure compliance with legal and ethical standards in research and experimentation.