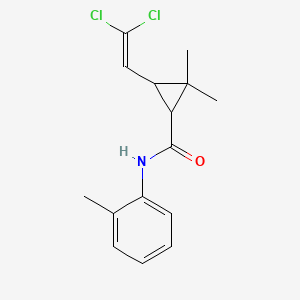
3-(2,2-dichloroethenyl)-2,2-dimethyl-N-(2-methylphenyl)cyclopropane-1-carboxamide
- Click on QUICK INQUIRY to receive a quote from our team of experts.
- With the quality product at a COMPETITIVE price, you can focus more on your research.
Overview
Description
3-(2,2-dichloroethenyl)-2,2-dimethyl-N-(2-methylphenyl)cyclopropane-1-carboxamide is a synthetic organic compound known for its unique chemical structure and properties This compound is characterized by a cyclopropane ring substituted with a dichloroethenyl group, a dimethyl group, and a carboxamide group attached to a 2-methylphenyl moiety
Preparation Methods
Synthetic Routes and Reaction Conditions
The synthesis of 3-(2,2-dichloroethenyl)-2,2-dimethyl-N-(2-methylphenyl)cyclopropane-1-carboxamide typically involves multiple steps, starting from readily available precursors. One common method involves the cyclopropanation of an appropriate alkene with a dichlorocarbene source, followed by functional group transformations to introduce the carboxamide and phenyl groups. The reaction conditions often require the use of strong bases, such as sodium hydride or potassium tert-butoxide, and solvents like tetrahydrofuran (THF) or dimethylformamide (DMF).
Industrial Production Methods
On an industrial scale, the production of this compound may involve optimized synthetic routes to ensure high yield and purity. This could include the use of continuous flow reactors, advanced purification techniques, and stringent quality control measures to meet regulatory standards.
Chemical Reactions Analysis
Types of Reactions
3-(2,2-dichloroethenyl)-2,2-dimethyl-N-(2-methylphenyl)cyclopropane-1-carboxamide undergoes various chemical reactions, including:
Oxidation: The compound can be oxidized using reagents like potassium permanganate or chromium trioxide to introduce oxygen-containing functional groups.
Reduction: Reduction reactions can be carried out using agents like lithium aluminum hydride or hydrogen gas in the presence of a catalyst to reduce specific functional groups.
Substitution: Nucleophilic substitution reactions can occur at the dichloroethenyl group, where nucleophiles like amines or thiols replace the chlorine atoms.
Common Reagents and Conditions
Oxidation: Potassium permanganate in aqueous or acidic medium.
Reduction: Lithium aluminum hydride in anhydrous ether or hydrogenation over a palladium catalyst.
Substitution: Nucleophiles such as sodium azide or thiourea in polar solvents like DMF.
Major Products
The major products formed from these reactions depend on the specific reagents and conditions used. For example, oxidation may yield carboxylic acids or ketones, while reduction can produce alcohols or amines.
Scientific Research Applications
Chemistry: Used as a building block for the synthesis of more complex molecules and as a reagent in organic transformations.
Biology: Investigated for its potential biological activity, including antimicrobial and anticancer properties.
Medicine: Explored as a candidate for drug development due to its unique structure and reactivity.
Industry: Utilized in the production of specialty chemicals and materials with specific properties.
Mechanism of Action
The mechanism of action of 3-(2,2-dichloroethenyl)-2,2-dimethyl-N-(2-methylphenyl)cyclopropane-1-carboxamide involves its interaction with molecular targets and pathways within biological systems. The compound may act by binding to specific enzymes or receptors, thereby modulating their activity. The exact pathways and targets can vary depending on the specific application and context of use.
Comparison with Similar Compounds
Similar Compounds
3-(2,2-dichloroethenyl)-2,2-dimethylcyclopropane-1-carboxamide: Lacks the phenyl group, resulting in different reactivity and applications.
2,2-dimethyl-N-(2-methylphenyl)cyclopropane-1-carboxamide:
3-(2,2-dichloroethenyl)-2,2-dimethylcyclopropane-1-carboxylic acid: Contains a carboxylic acid group instead of a carboxamide, leading to different reactivity and applications.
Uniqueness
The presence of both the dichloroethenyl and phenyl groups in 3-(2,2-dichloroethenyl)-2,2-dimethyl-N-(2-methylphenyl)cyclopropane-1-carboxamide imparts unique chemical properties, making it a versatile compound for various applications. Its ability to undergo diverse chemical reactions and its potential biological activity distinguish it from similar compounds.
Properties
Molecular Formula |
C15H17Cl2NO |
---|---|
Molecular Weight |
298.2 g/mol |
IUPAC Name |
3-(2,2-dichloroethenyl)-2,2-dimethyl-N-(2-methylphenyl)cyclopropane-1-carboxamide |
InChI |
InChI=1S/C15H17Cl2NO/c1-9-6-4-5-7-11(9)18-14(19)13-10(8-12(16)17)15(13,2)3/h4-8,10,13H,1-3H3,(H,18,19) |
InChI Key |
XDAHFTHPRAJJAG-UHFFFAOYSA-N |
Canonical SMILES |
CC1=CC=CC=C1NC(=O)C2C(C2(C)C)C=C(Cl)Cl |
Origin of Product |
United States |
Disclaimer and Information on In-Vitro Research Products
Please be aware that all articles and product information presented on BenchChem are intended solely for informational purposes. The products available for purchase on BenchChem are specifically designed for in-vitro studies, which are conducted outside of living organisms. In-vitro studies, derived from the Latin term "in glass," involve experiments performed in controlled laboratory settings using cells or tissues. It is important to note that these products are not categorized as medicines or drugs, and they have not received approval from the FDA for the prevention, treatment, or cure of any medical condition, ailment, or disease. We must emphasize that any form of bodily introduction of these products into humans or animals is strictly prohibited by law. It is essential to adhere to these guidelines to ensure compliance with legal and ethical standards in research and experimentation.