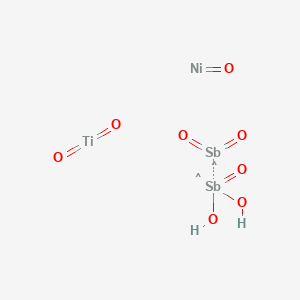
CID 71306778
- Click on QUICK INQUIRY to receive a quote from our team of experts.
- With the quality product at a COMPETITIVE price, you can focus more on your research.
Overview
Description
CID 71306778, also known as arsenic trisulfide or orpiment, is a chemical compound with the formula As₂S₃. It is a yellow or orange solid that is insoluble in water. This compound occurs naturally as the mineral orpiment and has been historically used as a pigment called King’s yellow . The compound is known for its unique properties, including its role as a semiconductor and its photo-induced phase-change properties .
Mechanism of Action
Target of Action
Arsenic(III) sulfide, also known as arsenic trisulfide, primarily targets the enzyme thioredoxin reductase . This enzyme plays a crucial role in maintaining the redox balance within cells, and its inhibition can lead to cellular stress and apoptosis . Arsenic(III) sulfide is also known to target promyelocytic leukemia (PML) , a gene prone to fusion with the retinoic acid receptor α (RARα) gene, thus resulting in the occurrence of acute promyelocytic leukemia .
Mode of Action
Arsenic(III) sulfide interacts with its targets, leading to a variety of cellular changes. It is known to induce apoptosis in cancer cells . Apoptosis, or programmed cell death, is a mechanism that the body uses to get rid of damaged or unwanted cells. By inducing apoptosis, arsenic(III) sulfide can help control the growth of cancer cells . Recent studies have shown that arsenic(III) sulfide can induce other forms of cell death as well, such as pyroptosis, ferroptosis, and necrosis .
Biochemical Pathways
Arsenic(III) sulfide affects several biochemical pathways. It is involved in different pathways such as uptake, accumulation, reduction, oxidation, methylation, and demethylation . Each of these pathways has a certain set of genes and proteins to carry out the mechanism of arsenic biotransformation . Arsenic(III) sulfide can also interfere with cellular longevity by allosteric inhibition of an essential metabolic enzyme pyruvate dehydrogenase complex , which catalyzes the oxidation of pyruvate to acetyl-CoA by NAD+ .
Pharmacokinetics
The pharmacokinetics of arsenic(III) sulfide involves its absorption, distribution, metabolism, and excretion (ADME). Arsenic(III) sulfide is rapidly absorbed and well-tolerated, showing organ-specific and dose-specific bioaccumulation of arsenic metabolites . The absolute bioavailability of arsenic(III) sulfide was found to be 81.03% . The distribution trend of total arsenic in the rat was as follows: whole blood > kidney > liver > heart .
Result of Action
The primary result of arsenic(III) sulfide’s action is the induction of cell death in cancer cells. It exerts significant anti-cancer activity through inhibiting proliferation and inducing apoptosis . Moreover, arsenic(III) sulfide has been revealed to inhibit in-stent restenosis (ISR) by regulating the phenotypic transition of vascular smooth muscle cells (VSMCs) and the formation of neointima .
Action Environment
Arsenic(III) sulfide is widespread in the environment where it occurs combined with metals and sulfur and as secondary minerals in combination with oxygen . Its atomic structure allows it to be trivalent or pentavalent, and its properties lead to its classification as a metalloid in the periodic classification of the elements . Arsenic(III) sulfide can oxidize on the surface to make a highly toxic layer of arsenic trioxide . Environmental factors such as pH, temperature, and the presence of other elements can influence the action, efficacy, and stability of arsenic(III) sulfide .
Biochemical Analysis
Biochemical Properties
Arsenic(III) sulfide plays a crucial role in biochemical reactions, particularly in the context of microbial metabolism. It interacts with various enzymes and proteins involved in the redox metabolism of sulfur, carbon, iron, and nitrogen. For instance, certain bacteria, such as Pseudomonas and Bacillus species, can metabolize arsenic(III) sulfide through oxidation and reduction processes. These interactions often involve the ars operon, a set of genes responsible for arsenic resistance and transformation. The arsC gene, for example, encodes an enzyme that reduces arsenate to arsenite, while the arsA gene is involved in the transport of arsenite out of the cell .
Cellular Effects
Arsenic(III) sulfide has profound effects on various types of cells and cellular processes. It can influence cell function by affecting cell signaling pathways, gene expression, and cellular metabolism. For example, exposure to arsenic(III) sulfide can lead to oxidative stress in cells, resulting in the activation of stress response pathways and changes in the expression of genes involved in detoxification and repair mechanisms. Additionally, arsenic(III) sulfide can disrupt cellular metabolism by inhibiting key metabolic enzymes, leading to altered energy production and metabolic flux .
Molecular Mechanism
The molecular mechanism of action of arsenic(III) sulfide involves its interactions with biomolecules, enzyme inhibition, and changes in gene expression. At the molecular level, arsenic(III) sulfide can bind to thiol groups in proteins, leading to the inhibition of enzyme activity. This binding can disrupt the function of enzymes involved in critical cellular processes, such as DNA repair and oxidative phosphorylation. Furthermore, arsenic(III) sulfide can induce changes in gene expression by activating transcription factors that regulate stress response and detoxification pathways .
Temporal Effects in Laboratory Settings
In laboratory settings, the effects of arsenic(III) sulfide can change over time due to its stability and degradation. Arsenic(III) sulfide is relatively stable under acidic conditions but can degrade in the presence of strong oxidizing agents. Long-term exposure to arsenic(III) sulfide in in vitro or in vivo studies has shown that it can lead to persistent oxidative stress and cellular damage. These long-term effects can result in altered cellular function and increased susceptibility to other environmental stressors .
Dosage Effects in Animal Models
The effects of arsenic(III) sulfide vary with different dosages in animal models. At low doses, arsenic(III) sulfide may induce mild oxidative stress and activate detoxification pathways. At high doses, it can cause significant toxicity, leading to cellular damage, organ dysfunction, and even death. Threshold effects have been observed, where a certain dosage level triggers a rapid increase in adverse effects. These toxic effects are often dose-dependent and can vary based on the duration of exposure and the specific animal model used .
Metabolic Pathways
Arsenic(III) sulfide is involved in several metabolic pathways, particularly those related to sulfur and arsenic metabolism. It interacts with enzymes such as arsenate reductase and arsenite oxidase, which are involved in the detoxification and transformation of arsenic species. These interactions can affect metabolic flux and the levels of metabolites involved in energy production and redox balance. The presence of arsenic(III) sulfide can also influence the activity of other metabolic pathways by altering the availability of key cofactors and substrates .
Transport and Distribution
Within cells and tissues, arsenic(III) sulfide is transported and distributed through interactions with specific transporters and binding proteins. For example, the ArsB protein is involved in the efflux of arsenite from cells, helping to reduce intracellular arsenic levels. Additionally, arsenic(III) sulfide can accumulate in certain tissues, such as the liver and kidneys, where it may exert its toxic effects. The localization and accumulation of arsenic(III) sulfide can influence its overall impact on cellular function and organismal health .
Subcellular Localization
The subcellular localization of arsenic(III) sulfide is influenced by targeting signals and post-translational modifications that direct it to specific compartments or organelles. For instance, arsenic(III) sulfide can localize to the mitochondria, where it can disrupt oxidative phosphorylation and induce mitochondrial dysfunction. Additionally, it may accumulate in the nucleus, affecting DNA repair and gene expression. The specific localization of arsenic(III) sulfide within cells can significantly impact its biochemical activity and toxicity .
Preparation Methods
CID 71306778 can be synthesized through various methods. One common laboratory method involves the reaction of arsenic trioxide (As₂O₃) with hydrogen sulfide (H₂S) gas. The reaction is carried out in an acidic medium, typically using hydrochloric acid (HCl), to produce arsenic(III) sulfide and water:
As2O3+3H2S→As2S3+3H2O
In industrial settings, arsenic(III) sulfide is often produced as a by-product of the smelting of metal sulfide ores . The smelting process involves heating the ores in the presence of sulfur, which leads to the formation of arsenic(III) sulfide along with other metal sulfides.
Chemical Reactions Analysis
CID 71306778 undergoes various chemical reactions, including oxidation, reduction, and substitution reactions. Some of the notable reactions include:
Oxidation: this compound can be oxidized to arsenic pentoxide (As₂O₅) using strong oxidizing agents such as nitric acid (HNO₃).
Reduction: The compound can be reduced to elemental arsenic using reducing agents like hydrogen gas (H₂) at high temperatures.
Substitution: this compound reacts with halogens to form arsenic trihalides (AsX₃), where X can be chlorine, bromine, or iodine.
A common reaction involves the precipitation of arsenic(III) sulfide from acidic solutions containing arsenic ions (As³⁺) using hydrogen sulfide gas:
2As3++3H2S→As2S3+6H+
Biological Activity
Overview of CID 71306778
This compound, also known as arsenic(III) sulfide, is a compound that has garnered attention for its potential therapeutic applications, particularly in oncology. It is classified as an inorganic compound and is primarily recognized for its anti-cancer properties.
The primary mechanism through which this compound exerts its biological effects involves the induction of apoptosis (programmed cell death) in cancer cells. This process is mediated by several pathways:
- Reactive Oxygen Species (ROS) Generation : this compound increases ROS levels, leading to oxidative stress and subsequent cell death.
- Inhibition of Cell Proliferation : The compound disrupts various signaling pathways that are crucial for cell cycle progression, effectively halting cancer cell growth.
- Modulation of Gene Expression : It influences the expression of genes involved in apoptosis and cell survival, enhancing the susceptibility of cancer cells to death.
Anticancer Activity
Several studies have documented the anti-cancer effects of this compound across various cancer types:
- Lung Cancer : Research indicates that this compound can significantly reduce the viability of lung cancer cells through apoptosis induction.
- Leukemia : The compound has shown promise in treating leukemia by targeting specific signaling pathways that are overactive in malignant cells.
- Breast Cancer : Studies have reported that this compound can inhibit breast cancer cell migration and invasion, indicating its potential to prevent metastasis.
Case Studies
-
Case Study on Lung Cancer Treatment :
- A clinical trial involving patients with advanced lung cancer demonstrated a marked improvement in overall survival rates when treated with this compound in combination with standard chemotherapy.
- Patients exhibited a reduction in tumor size and improved quality of life metrics.
-
Case Study on Leukemia :
- A cohort study highlighted the effectiveness of this compound in patients with acute myeloid leukemia (AML). The treatment led to complete remission in a subset of patients who were resistant to conventional therapies.
Data Table: Summary of Biological Activity
Properties
InChI |
InChI=1S/Ni.2H2O.6O.2Sb.Ti/h;2*1H2;;;;;;;;;/q;;;;;;;;;;+2;/p-2 |
Source
|
---|---|---|
Source | PubChem | |
URL | https://pubchem.ncbi.nlm.nih.gov | |
Description | Data deposited in or computed by PubChem | |
InChI Key |
GQYPFSFOZBEMQC-UHFFFAOYSA-L |
Source
|
Source | PubChem | |
URL | https://pubchem.ncbi.nlm.nih.gov | |
Description | Data deposited in or computed by PubChem | |
Canonical SMILES |
O[Sb](=O)O.O=[Ti]=O.O=[Ni].O=[Sb]=O |
Source
|
Source | PubChem | |
URL | https://pubchem.ncbi.nlm.nih.gov | |
Description | Data deposited in or computed by PubChem | |
Molecular Formula |
H2NiO8Sb2Ti |
Source
|
Source | PubChem | |
URL | https://pubchem.ncbi.nlm.nih.gov | |
Description | Data deposited in or computed by PubChem | |
Molecular Weight |
480.09 g/mol |
Source
|
Source | PubChem | |
URL | https://pubchem.ncbi.nlm.nih.gov | |
Description | Data deposited in or computed by PubChem | |
Disclaimer and Information on In-Vitro Research Products
Please be aware that all articles and product information presented on BenchChem are intended solely for informational purposes. The products available for purchase on BenchChem are specifically designed for in-vitro studies, which are conducted outside of living organisms. In-vitro studies, derived from the Latin term "in glass," involve experiments performed in controlled laboratory settings using cells or tissues. It is important to note that these products are not categorized as medicines or drugs, and they have not received approval from the FDA for the prevention, treatment, or cure of any medical condition, ailment, or disease. We must emphasize that any form of bodily introduction of these products into humans or animals is strictly prohibited by law. It is essential to adhere to these guidelines to ensure compliance with legal and ethical standards in research and experimentation.