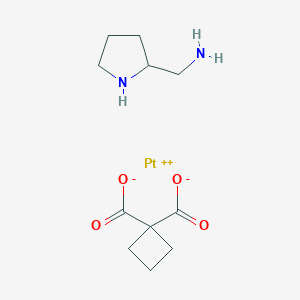
Cyclobutane-1,1-dicarboxylate;platinum(2+);pyrrolidin-2-ylmethanamine
- Click on QUICK INQUIRY to receive a quote from our team of experts.
- With the quality product at a COMPETITIVE price, you can focus more on your research.
Overview
Description
Cyclobutane-1,1-dicarboxylate;platinum(2+);pyrrolidin-2-ylmethanamine is a compound with the molecular formula C11H18N2O4Pt and a molecular weight of 437.4 g/mol. It is a nonnephrotoxic analogue of cisplatin currently undergoing clinical evaluation .
Synthesis Analysis
Carboplatin (cis diammine cyclobutane dicarboxylate platinum (II)) is a second-generation drug that is less toxic than cisplatin, allowing for high dosages . In the first stage, 1,3-Cyclobutane dicarboxylic acid, a key intermediate for the preparation of carboplatin, is synthesized in good yields using different methodologies to achieve high purity. Then, carboplatin is synthesized from the reaction of the prepared ligand with cisplatin .Molecular Structure Analysis
The molecular structure of this compound includes a cyclobutane ring with two carboxylate groups attached to the same carbon atom, a platinum(2+) ion, and a pyrrolidin-2-ylmethanamine group .Chemical Reactions Analysis
The major route of elimination of this compound is renal, with 65% of the platinum administered being excreted in the urine within 24 hours, with 32% of the dose excreted as unchanged CBDCA .Physical And Chemical Properties Analysis
This compound has a molecular weight of 437.4 g/mol. It is a stable complex with a half-life of 37°; plasma, 30 hr, and urine (range), 20 to 460 hr .Scientific Research Applications
Oncology: Anticancer Drug Development
The compound has been studied for its potential as a nonnephrotoxic analogue of cisplatin . It’s undergoing clinical evaluation for its pharmacokinetic properties, which include a stable complex formation and a significant renal excretion as unchanged CBDCA, making it a promising candidate for anticancer drug development .
Pharmacokinetics: Renal Function Impact
Research has shown that the compound’s pharmacokinetics are not dose-dependent and that its plasma clearance correlates with glomerular filtration rates . This suggests its safe application in patients with varying degrees of renal function, expanding its therapeutic window .
Chemotherapy: Side Effect Reduction
The compound’s stability and nonnephrotoxic nature suggest it could reduce the side effects commonly associated with platinum-based chemotherapy drugs . This could lead to improved patient quality of life during treatment.
Cancer Cell Line Studies: In Vitro Efficacy
Synthesized analogues of the compound have demonstrated potent anticancer activity in vitro against various cancer cell lines, including lung carcinoma (A549), ovarian carcinoma (SK-OV-3), and colon cancer cell (HT-29) lines . This highlights its potential for broad-spectrum anticancer applications.
Acute Toxicity: Comparative Analysis
Preliminary studies on the acute toxicity of the compound’s derivatives have shown a similar level of toxicity as carboplatin and lower toxicity than cisplatin in vivo . This indicates a safer profile for clinical use.
Water Solubility: Drug Formulation
The compound and its derivatives have been characterized by satisfactory water solubility . This property is crucial for drug formulation, as it facilitates the preparation of aqueous solutions for intravenous administration.
Safety And Hazards
Future Directions
properties
{ "Design of the Synthesis Pathway": "The synthesis of Cyclobutane-1,1-dicarboxylate;platinum(2+);pyrrolidin-2-ylmethanamine can be achieved through a multi-step process that involves the formation of intermediate compounds. The first step involves the synthesis of cyclobutane-1,1-dicarboxylic acid, which is then converted to its corresponding acid chloride. The acid chloride is then reacted with pyrrolidin-2-ylmethanamine to form the amide intermediate. Finally, the platinum(2+) complex is added to the amide intermediate to form the desired compound.", "Starting Materials": [ "Butadiene", "Ozone", "Hydrogen peroxide", "Methanol", "Sodium hydroxide", "Thionyl chloride", "Pyrrolidin-2-ylmethanamine", "Platinum(2+) chloride" ], "Reaction": [ "Step 1: Butadiene is oxidized with ozone to form cyclobutane-1,1-diol.", "Step 2: Cyclobutane-1,1-diol is oxidized with hydrogen peroxide in the presence of methanol and sodium hydroxide to form cyclobutane-1,1-dicarboxylic acid.", "Step 3: Cyclobutane-1,1-dicarboxylic acid is converted to its corresponding acid chloride using thionyl chloride.", "Step 4: The acid chloride is reacted with pyrrolidin-2-ylmethanamine to form the amide intermediate.", "Step 5: Platinum(2+) chloride is added to the amide intermediate to form the desired compound, Cyclobutane-1,1-dicarboxylate;platinum(2+);pyrrolidin-2-ylmethanamine." ] } | |
CAS RN |
103775-75-3 |
Product Name |
Cyclobutane-1,1-dicarboxylate;platinum(2+);pyrrolidin-2-ylmethanamine |
Molecular Formula |
C11H18N2O4Pt |
Molecular Weight |
437.4 g/mol |
IUPAC Name |
cyclobutane-1,1-dicarboxylate;platinum(2+);pyrrolidin-2-ylmethanamine |
InChI |
InChI=1S/C6H8O4.C5H12N2.Pt/c7-4(8)6(5(9)10)2-1-3-6;6-4-5-2-1-3-7-5;/h1-3H2,(H,7,8)(H,9,10);5,7H,1-4,6H2;/q;;+2/p-2 |
InChI Key |
XXUHLUDUCZQMDI-UHFFFAOYSA-L |
Isomeric SMILES |
C1C[C@@H](NC1)CN.C1CC(C1)(C(=O)[O-])C(=O)[O-].[Pt+2] |
SMILES |
C1CC(NC1)CN.C1CC(C1)(C(=O)[O-])C(=O)[O-].[Pt+2] |
Canonical SMILES |
C1CC(NC1)CN.C1CC(C1)(C(=O)[O-])C(=O)[O-].[Pt+2] |
synonyms |
1,1-cyclobutanedicarboxylato(2-aminomethylpyrrolidine)platinum(II) 2-aminomethylpyrrolidine(1,1-cyclobutanedicarboxylatato)platinum II DW A 2114R DWA 2114 DWA 2114R DWA-2114 DWA-2114R |
Origin of Product |
United States |
Disclaimer and Information on In-Vitro Research Products
Please be aware that all articles and product information presented on BenchChem are intended solely for informational purposes. The products available for purchase on BenchChem are specifically designed for in-vitro studies, which are conducted outside of living organisms. In-vitro studies, derived from the Latin term "in glass," involve experiments performed in controlled laboratory settings using cells or tissues. It is important to note that these products are not categorized as medicines or drugs, and they have not received approval from the FDA for the prevention, treatment, or cure of any medical condition, ailment, or disease. We must emphasize that any form of bodily introduction of these products into humans or animals is strictly prohibited by law. It is essential to adhere to these guidelines to ensure compliance with legal and ethical standards in research and experimentation.