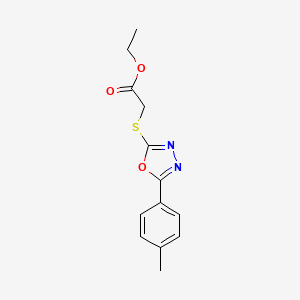
Ethyl 2-((5-(p-tolyl)-1,3,4-oxadiazol-2-yl)thio)acetate
- Click on QUICK INQUIRY to receive a quote from our team of experts.
- With the quality product at a COMPETITIVE price, you can focus more on your research.
Overview
Description
Ethyl 2-((5-(p-tolyl)-1,3,4-oxadiazol-2-yl)thio)acetate is a heterocyclic compound that contains an oxadiazole ring. This compound is of interest due to its potential applications in various fields such as medicinal chemistry, organic synthesis, and materials science. The presence of the oxadiazole ring imparts unique chemical and physical properties to the compound, making it a valuable target for research and development.
Preparation Methods
Synthetic Routes and Reaction Conditions
The synthesis of Ethyl 2-((5-(p-tolyl)-1,3,4-oxadiazol-2-yl)thio)acetate typically involves the reaction of p-tolyl hydrazine with carbon disulfide and ethyl chloroacetate in the presence of a base. The reaction proceeds through the formation of a thiosemicarbazide intermediate, which then cyclizes to form the oxadiazole ring. The reaction conditions generally include refluxing the reactants in an appropriate solvent such as ethanol or methanol .
Industrial Production Methods
While specific industrial production methods for this compound are not well-documented, the general approach would involve scaling up the laboratory synthesis. This would include optimizing reaction conditions to maximize yield and purity, as well as implementing efficient purification techniques such as recrystallization or chromatography.
Chemical Reactions Analysis
Types of Reactions
Ethyl 2-((5-(p-tolyl)-1,3,4-oxadiazol-2-yl)thio)acetate can undergo various chemical reactions, including:
Oxidation: The compound can be oxidized to form sulfoxides or sulfones.
Reduction: Reduction reactions can convert the oxadiazole ring to other heterocyclic structures.
Substitution: The ethyl ester group can be substituted with other functional groups through nucleophilic substitution reactions.
Common Reagents and Conditions
Oxidation: Common oxidizing agents include hydrogen peroxide and m-chloroperbenzoic acid.
Reduction: Reducing agents such as lithium aluminum hydride or sodium borohydride are typically used.
Substitution: Nucleophiles such as amines or alcohols can be used under basic conditions to substitute the ethyl ester group.
Major Products
The major products formed from these reactions depend on the specific conditions and reagents used. For example, oxidation can yield sulfoxides or sulfones, while substitution reactions can produce a variety of ester derivatives.
Scientific Research Applications
Ethyl 2-((5-(p-tolyl)-1,3,4-oxadiazol-2-yl)thio)acetate has several scientific research applications:
Medicinal Chemistry: The compound is studied for its potential as an antimicrobial, anticancer, and anti-inflammatory agent.
Organic Synthesis: It serves as a building block for the synthesis of more complex molecules.
Materials Science: The unique properties of the oxadiazole ring make it useful in the development of new materials with specific electronic or optical properties.
Mechanism of Action
The mechanism of action of Ethyl 2-((5-(p-tolyl)-1,3,4-oxadiazol-2-yl)thio)acetate involves its interaction with specific molecular targets. In medicinal applications, it may inhibit the activity of certain enzymes or receptors, leading to its therapeutic effects. The exact molecular pathways depend on the specific application and target .
Comparison with Similar Compounds
Similar Compounds
- Ethyl 2-(2-(2-(5-(1-(4-chlorophenoxy)-ethyl)-4-phenyl-4H-1,2,4-triazol-3-ylthio)acetamido)thiazol-4-yl)acetate
- 5-(2-substituted–1,3-thiazol-5-yl)-2-alkoxybenzamides
Uniqueness
Ethyl 2-((5-(p-tolyl)-1,3,4-oxadiazol-2-yl)thio)acetate is unique due to the presence of the oxadiazole ring, which imparts distinct chemical and physical properties. This makes it particularly valuable in applications where stability and specific reactivity are required .
Properties
Molecular Formula |
C13H14N2O3S |
---|---|
Molecular Weight |
278.33 g/mol |
IUPAC Name |
ethyl 2-[[5-(4-methylphenyl)-1,3,4-oxadiazol-2-yl]sulfanyl]acetate |
InChI |
InChI=1S/C13H14N2O3S/c1-3-17-11(16)8-19-13-15-14-12(18-13)10-6-4-9(2)5-7-10/h4-7H,3,8H2,1-2H3 |
InChI Key |
ORJVVXIRGUDCAZ-UHFFFAOYSA-N |
Canonical SMILES |
CCOC(=O)CSC1=NN=C(O1)C2=CC=C(C=C2)C |
Origin of Product |
United States |
Disclaimer and Information on In-Vitro Research Products
Please be aware that all articles and product information presented on BenchChem are intended solely for informational purposes. The products available for purchase on BenchChem are specifically designed for in-vitro studies, which are conducted outside of living organisms. In-vitro studies, derived from the Latin term "in glass," involve experiments performed in controlled laboratory settings using cells or tissues. It is important to note that these products are not categorized as medicines or drugs, and they have not received approval from the FDA for the prevention, treatment, or cure of any medical condition, ailment, or disease. We must emphasize that any form of bodily introduction of these products into humans or animals is strictly prohibited by law. It is essential to adhere to these guidelines to ensure compliance with legal and ethical standards in research and experimentation.