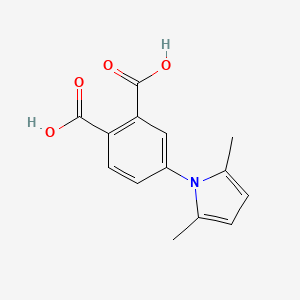
4-(2,5-dimethylpyrrol-1-yl)phthalic Acid
- Click on QUICK INQUIRY to receive a quote from our team of experts.
- With the quality product at a COMPETITIVE price, you can focus more on your research.
Overview
Description
4-(2,5-Dimethylpyrrol-1-yl)phthalic acid is a derivative of phthalic acid featuring a 2,5-dimethylpyrrole substituent at the 4-position of the benzene ring. This compound has garnered attention in medicinal chemistry due to its antimicrobial, antifungal, and antitubercular activities . Its structure enables interactions with biological targets, particularly enzymes such as those involved in bacterial cell wall synthesis or fungal metabolism. Key interactions involve hydrogen bonding and hydrophobic contacts with residues like ARG60, ARG32, and GLN28, as observed in molecular docking studies .
Preparation Methods
Synthetic Routes and Reaction Conditions
The synthesis of 4-(2,5-dimethyl-1H-pyrrol-1-yl)benzene-1,2-dicarboxylic acid can be achieved through several methods. One common approach involves the reaction of 2,5-dimethylpyrrole with benzene-1,2-dicarboxylic anhydride under acidic conditions. The reaction typically takes place in a solvent such as acetic acid, and the mixture is heated to facilitate the formation of the desired product .
Industrial Production Methods
In an industrial setting, the production of this compound may involve the use of continuous flow reactors to ensure consistent quality and yield. The reaction conditions are optimized to maximize the efficiency of the process, and advanced purification techniques such as recrystallization or chromatography are employed to obtain the pure compound .
Chemical Reactions Analysis
Types of Reactions
4-(2,5-dimethyl-1H-pyrrol-1-yl)benzene-1,2-dicarboxylic acid undergoes various chemical reactions, including:
Oxidation: The compound can be oxidized to form corresponding quinones or other oxidized derivatives.
Reduction: Reduction reactions can convert the carboxylic acid groups to alcohols or other reduced forms.
Substitution: Electrophilic substitution reactions can occur on the benzene ring, leading to the formation of various substituted derivatives.
Common Reagents and Conditions
Oxidation: Common oxidizing agents include potassium permanganate (KMnO4) and chromium trioxide (CrO3).
Reduction: Reducing agents such as lithium aluminum hydride (LiAlH4) or sodium borohydride (NaBH4) are typically used.
Substitution: Reagents like halogens (e.g., bromine) or nitrating agents (e.g., nitric acid) are employed under controlled conditions[][4].
Major Products Formed
The major products formed from these reactions depend on the specific conditions and reagents used. For example, oxidation may yield quinones, while reduction can produce alcohols. Substitution reactions can lead to halogenated or nitrated derivatives .
Scientific Research Applications
4-(2,5-dimethyl-1H-pyrrol-1-yl)benzene-1,2-dicarboxylic acid has several applications in scientific research:
Chemistry: It is used as a building block for the synthesis of more complex organic molecules and materials.
Biology: The compound has been studied for its potential biological activities, including antiproliferative effects on certain cell lines.
Medicine: Research is ongoing to explore its potential as a therapeutic agent for various diseases.
Industry: It is used in the development of new materials with specific properties, such as polymers and dyes.
Mechanism of Action
The mechanism by which 4-(2,5-dimethyl-1H-pyrrol-1-yl)benzene-1,2-dicarboxylic acid exerts its effects involves interactions with specific molecular targets and pathways. For example, it may inhibit certain enzymes or receptors, leading to changes in cellular processes. The exact molecular targets and pathways can vary depending on the specific application and context .
Comparison with Similar Compounds
Comparison with Structurally Similar Compounds
Structural and Functional Group Analysis
The table below compares 4-(2,5-dimethylpyrrol-1-yl)phthalic acid with structurally related compounds, emphasizing molecular weight, biological activity, and binding interactions:
Compound Name | Structure | Molecular Weight (g/mol) | Biological Activity | Key Interactions/Applications | References |
---|---|---|---|---|---|
This compound | Phthalic acid with 4-position 2,5-dimethylpyrrole substituent | ~245 (estimated) | Antimicrobial, Antifungal | Binds ARG60, ARG32, GLN28 residues | [4, 8] |
Novo4j | 4-[2,4-bis-(3-nitrobenzoylamino)phenoxy]phthalic acid | ~500 (estimated) | Glycogen phosphorylase inhibition | Allosteric site binding (2QN1/2QN2) | [1] |
4-(3-Carboxypropanamido)phthalic Acid (10) | Phthalic acid with 4-position carboxypropanamido group | ~285 (estimated) | Enzyme ligand (potential) | Carboxyl-metal coordination (MOFs) | [2, 3] |
[4-(2,5-Dimethylpyrrol-1-yl)phenyl]acetic Acid | Acetic acid derivative with 4-position 2,5-dimethylpyrrole substituent | 229.279 | Unknown (structural analog) | Similar pyrrole hydrophobicity | [5] |
Physicochemical Properties
- Solubility : The carboxyalkylamido substituents in compounds 10 and 11 improve aqueous solubility compared to the hydrophobic dimethylpyrrole group in the target compound, which may limit bioavailability .
Key Research Findings
- Docking Studies: The target compound exhibits consensus docking scores (4.29–6.09) comparable to reference ligands, suggesting strong binding to microbial enzymes. Novo4j, however, shows variability in docking results between software tools, underscoring the need for experimental validation .
- Structure-Activity Relationship (SAR) : The 2,5-dimethylpyrrole group is critical for antimicrobial activity, while nitro or carboxyalkylamido substituents redirect functionality toward enzyme inhibition or material science applications .
Q & A
Q. Basic: What are the recommended experimental design strategies for synthesizing 4-(2,5-dimethylpyrrol-1-yl)phthalic acid with high purity?
Methodological Answer:
A factorial design approach is recommended to optimize reaction parameters (e.g., temperature, stoichiometry, catalyst loading). Use statistical methods like Design of Experiments (DoE) to minimize trial-and-error experimentation. For example:
- Factors to vary : Reaction time (6–24 hrs), solvent polarity (DMF vs. THF), and protecting group strategy for the pyrrole moiety.
- Response variables : Yield, purity (via HPLC/LC-MS), and crystallinity (XRD).
Quantum chemical calculations (e.g., DFT) can predict favorable reaction pathways, while in-situ IR spectroscopy monitors intermediate formation .
Q. Advanced: How can computational methods resolve contradictions in spectroscopic data for this compound’s tautomeric forms?
Methodological Answer:
Conflicting NMR or IR data may arise from tautomerism or dynamic equilibria. Use multiconfigurational quantum mechanics (MC-QM) to model possible tautomers. Validate with:
- Variable-temperature NMR to detect slow-exchange tautomers.
- Solvent-dependent UV-Vis spectroscopy to identify solvent-stabilized conformers.
Compare computed vs. experimental chemical shifts (δ ppm) and coupling constants (J values) to assign dominant tautomers .
Q. Basic: What analytical techniques are most reliable for characterizing this compound?
Methodological Answer:
- NMR (¹H/¹³C/DEPT-135) : Assign aromatic protons (δ 6.8–8.2 ppm) and pyrrole methyl groups (δ 2.1–2.5 ppm).
- High-resolution mass spectrometry (HRMS) : Confirm molecular ion ([M+H]⁺) with <2 ppm error.
- X-ray crystallography : Resolve steric effects from the dimethylpyrrole substituents.
- HPLC-PDA : Assess purity (>98%) using a C18 column (MeCN/H2O + 0.1% TFA) .
Q. Advanced: How can reaction path sampling (RPS) improve the scalability of its synthesis?
Methodological Answer:
RPS identifies bottlenecks in scale-up by simulating reaction trajectories. Steps:
Microkinetic modeling : Predict rate-limiting steps (e.g., acid-catalyzed cyclization).
Inert gas sparging : Mitigate oxidation side reactions during large-batch runs.
Flow chemistry : Enhance heat/mass transfer for exothermic steps (e.g., phthalic anhydride coupling).
Validate with pilot-scale experiments using inline PAT (Process Analytical Technology) tools .
Q. Basic: What are the stability challenges for this compound under ambient storage conditions?
Methodological Answer:
The compound is prone to hydrolysis due to the ester-like linkage between pyrrole and phthalic acid. Mitigation strategies:
- Storage : Argon atmosphere, desiccated at −20°C.
- Stability testing : Accelerated degradation studies (40°C/75% RH for 4 weeks) with LC-MS to track decomposition products (e.g., free phthalic acid).
- Stabilizers : Add antioxidants (e.g., BHT at 0.01% w/w) to inhibit radical-mediated degradation .
Q. Advanced: How can machine learning (ML) models predict its reactivity in multicomponent reactions?
Methodological Answer:
Train ML models on existing reaction databases (e.g., Reaxys) using descriptors like:
- Electronic parameters : HOMO/LUMO energies (from DFT).
- Steric parameters : Tolman cone angle for the pyrrole substituents.
Validate predictions via high-throughput screening (HTS) in 96-well plates. Prioritize reactions with >80% predicted yield and <10% side-product formation .
Q. Basic: What are the key considerations for designing a catalytic system for its derivatization?
Methodological Answer:
- Catalyst selection : Lewis acids (e.g., Sc(OTf)₃) for electrophilic substitutions; Pd/C for hydrogenolysis.
- Solvent compatibility : Avoid protic solvents (e.g., H2O) to prevent ester hydrolysis.
- Protecting groups : Use Boc for the phthalic acid carboxyl groups during pyrrole functionalization.
Monitor regioselectivity via NOESY NMR to confirm substitution patterns .
Q. Advanced: How does steric hindrance from the dimethylpyrrole group affect its supramolecular assembly?
Methodological Answer:
Use single-crystal XRD to analyze packing motifs. The dimethyl groups disrupt π-π stacking, favoring hydrogen-bonded dimers via phthalic acid carboxyls. Quantify using:
- Hirshfeld surface analysis : Map intermolecular contacts (e.g., O–H···O vs. C–H···π).
- DFT-based lattice energy calculations : Compare stabilization energies of observed vs. hypothetical polymorphs .
Q. Basic: What solvent systems are optimal for its recrystallization?
Methodological Answer:
Screen solvent pairs (e.g., EtOAc/hexane, MeOH/H2O) using a ternary phase diagram. Ideal conditions:
- High solubility at elevated temps : 50 mg/mL in DMF at 80°C.
- Low solubility at RT : <5 mg/mL in EtOH.
Characterize crystals via DSC to confirm melting point (mp ~215–220°C) and absence of solvates .
Q. Advanced: How can isotopic labeling (e.g., ¹³C) elucidate its metabolic fate in biological studies?
Methodological Answer:
Synthesize ¹³C-labeled analogs at the phthalic acid carboxyl positions. Track using:
Properties
CAS No. |
313701-80-3 |
---|---|
Molecular Formula |
C14H13NO4 |
Molecular Weight |
259.26 g/mol |
IUPAC Name |
4-(2,5-dimethylpyrrol-1-yl)phthalic acid |
InChI |
InChI=1S/C14H13NO4/c1-8-3-4-9(2)15(8)10-5-6-11(13(16)17)12(7-10)14(18)19/h3-7H,1-2H3,(H,16,17)(H,18,19) |
InChI Key |
UQFYRZIMRBEYDD-UHFFFAOYSA-N |
Canonical SMILES |
CC1=CC=C(N1C2=CC(=C(C=C2)C(=O)O)C(=O)O)C |
Origin of Product |
United States |
Disclaimer and Information on In-Vitro Research Products
Please be aware that all articles and product information presented on BenchChem are intended solely for informational purposes. The products available for purchase on BenchChem are specifically designed for in-vitro studies, which are conducted outside of living organisms. In-vitro studies, derived from the Latin term "in glass," involve experiments performed in controlled laboratory settings using cells or tissues. It is important to note that these products are not categorized as medicines or drugs, and they have not received approval from the FDA for the prevention, treatment, or cure of any medical condition, ailment, or disease. We must emphasize that any form of bodily introduction of these products into humans or animals is strictly prohibited by law. It is essential to adhere to these guidelines to ensure compliance with legal and ethical standards in research and experimentation.