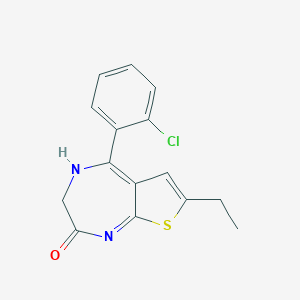
Desmethylclotiazepam
Overview
Description
Desmethylclotiazepam is a thienodiazepine compound and an active metabolite of clotiazepam. It is known for its anxiolytic, anticonvulsant, and muscle relaxant properties . The compound has a molecular formula of C15H13ClN2OS and a molar mass of 304.8 g/mol .
Mechanism of Action
Target of Action
Desmethylclotiazepam, also known as N-Desmethyl Clotiazepam, is an active metabolite of clotiazepam . It primarily targets the GABA (A) Receptor , a key player in inhibitory neurotransmission in the central nervous system . This receptor is crucial for maintaining the balance between neuronal excitation and inhibition.
Mode of Action
This compound acts as a positive allosteric modulator at the GABA (A) Receptor . It binds to the benzodiazepine site of the GABA (A) receptor, enhancing the inhibitory effect of GABA . This results in an increase in the frequency of opening of the chloride channel and penetration of chloride ions through the ionophore . The increased membrane polarization decreases the probability of neuron discharge, leading to sedative and anxiolytic effects .
Biochemical Pathways
The enhancement of GABAergic inhibition can lead to downstream effects such as reduced neuronal excitability and decreased anxiety .
Pharmacokinetics
It is known that clotiazepam, the parent compound, has a bioavailability of approximately 90% and is metabolized in the liver . The elimination half-life of clotiazepam is around 4 hours . As this compound is a metabolite of clotiazepam, it is likely to share similar pharmacokinetic properties .
Result of Action
The molecular and cellular effects of this compound’s action primarily involve the enhancement of GABAergic inhibition. This leads to a decrease in neuronal excitability, which can result in anxiolytic, anticonvulsant, and sedative effects .
Biochemical Analysis
Biochemical Properties
Desmethylclotiazepam, as a metabolite of clotiazepam, is likely to share similar biochemical properties with its parent compound. Clotiazepam is known to bind to the benzodiazepine site of the GABA A receptor, where it acts as a full agonist . This action results in an enhanced GABA inhibitory effect at the GABA A receptor, which leads to the pharmacological effects of clotiazepam . Therefore, it is plausible that this compound may interact with similar enzymes, proteins, and other biomolecules.
Cellular Effects
Given its relationship to clotiazepam, it may influence cell function by modulating GABAergic neurotransmission . This could potentially impact cell signaling pathways, gene expression, and cellular metabolism.
Molecular Mechanism
This compound, being a metabolite of clotiazepam, may share a similar mechanism of action. Clotiazepam acts at the benzodiazepine receptors (BZD), increasing the frequency of opening of the channel chlorinates and penetration of the ions chlorinates through the ionophore . This increase in membrane polarization decreases the probability of discharge of neurons .
Metabolic Pathways
This compound is a metabolite of clotiazepam, which is metabolized in the liver
Preparation Methods
Synthetic Routes and Reaction Conditions: Desmethylclotiazepam can be synthesized through the demethylation of clotiazepam. The process involves the removal of a methyl group from the parent compound, clotiazepam, under specific reaction conditions .
Industrial Production Methods: Industrial production of this compound typically involves large-scale synthesis using optimized reaction conditions to ensure high yield and purity. The process may include steps such as crystallization and purification to obtain the final product .
Types of Reactions:
Oxidation: this compound can undergo oxidation reactions, leading to the formation of various oxidized metabolites.
Reduction: The compound can also be reduced under specific conditions to yield reduced forms.
Substitution: this compound can participate in substitution reactions, where functional groups are replaced by other groups.
Common Reagents and Conditions:
Oxidation: Common oxidizing agents include hydrogen peroxide and potassium permanganate.
Reduction: Reducing agents such as lithium aluminum hydride can be used.
Substitution: Various halogenating agents and nucleophiles are employed in substitution reactions.
Major Products Formed: The major products formed from these reactions depend on the specific reagents and conditions used. For example, oxidation may yield hydroxylated metabolites, while reduction can produce desmethylated derivatives .
Scientific Research Applications
Desmethylclotiazepam is widely used in scientific research due to its pharmacological properties. It serves as an analytical reference standard in forensic and pharmaceutical research. The compound is also studied for its potential therapeutic applications in treating anxiety disorders, epilepsy, and muscle spasms .
Comparison with Similar Compounds
Clotiazepam: The parent compound from which desmethylclotiazepam is derived.
Diazepam: Another benzodiazepine with anxiolytic and anticonvulsant properties.
Lorazepam: A benzodiazepine with similar therapeutic uses but different metabolic pathways and potency.
Uniqueness: this compound is unique due to its specific metabolic pathway and the absence of a methyl group compared to clotiazepam. This structural difference can influence its pharmacokinetics and pharmacodynamics, making it a valuable compound for research and therapeutic applications .
Properties
IUPAC Name |
5-(2-chlorophenyl)-7-ethyl-1,3-dihydrothieno[2,3-e][1,4]diazepin-2-one | |
---|---|---|
Source | PubChem | |
URL | https://pubchem.ncbi.nlm.nih.gov | |
Description | Data deposited in or computed by PubChem | |
InChI |
InChI=1S/C15H13ClN2OS/c1-2-9-7-11-14(10-5-3-4-6-12(10)16)17-8-13(19)18-15(11)20-9/h3-7H,2,8H2,1H3,(H,18,19) | |
Source | PubChem | |
URL | https://pubchem.ncbi.nlm.nih.gov | |
Description | Data deposited in or computed by PubChem | |
InChI Key |
NDWDXZCLEWQSMO-UHFFFAOYSA-N | |
Source | PubChem | |
URL | https://pubchem.ncbi.nlm.nih.gov | |
Description | Data deposited in or computed by PubChem | |
Canonical SMILES |
CCC1=CC2=C(S1)NC(=O)CN=C2C3=CC=CC=C3Cl | |
Source | PubChem | |
URL | https://pubchem.ncbi.nlm.nih.gov | |
Description | Data deposited in or computed by PubChem | |
Molecular Formula |
C15H13ClN2OS | |
Source | PubChem | |
URL | https://pubchem.ncbi.nlm.nih.gov | |
Description | Data deposited in or computed by PubChem | |
Molecular Weight |
304.8 g/mol | |
Source | PubChem | |
URL | https://pubchem.ncbi.nlm.nih.gov | |
Description | Data deposited in or computed by PubChem | |
Retrosynthesis Analysis
AI-Powered Synthesis Planning: Our tool employs the Template_relevance Pistachio, Template_relevance Bkms_metabolic, Template_relevance Pistachio_ringbreaker, Template_relevance Reaxys, Template_relevance Reaxys_biocatalysis model, leveraging a vast database of chemical reactions to predict feasible synthetic routes.
One-Step Synthesis Focus: Specifically designed for one-step synthesis, it provides concise and direct routes for your target compounds, streamlining the synthesis process.
Accurate Predictions: Utilizing the extensive PISTACHIO, BKMS_METABOLIC, PISTACHIO_RINGBREAKER, REAXYS, REAXYS_BIOCATALYSIS database, our tool offers high-accuracy predictions, reflecting the latest in chemical research and data.
Strategy Settings
Precursor scoring | Relevance Heuristic |
---|---|
Min. plausibility | 0.01 |
Model | Template_relevance |
Template Set | Pistachio/Bkms_metabolic/Pistachio_ringbreaker/Reaxys/Reaxys_biocatalysis |
Top-N result to add to graph | 6 |
Feasible Synthetic Routes
Disclaimer and Information on In-Vitro Research Products
Please be aware that all articles and product information presented on BenchChem are intended solely for informational purposes. The products available for purchase on BenchChem are specifically designed for in-vitro studies, which are conducted outside of living organisms. In-vitro studies, derived from the Latin term "in glass," involve experiments performed in controlled laboratory settings using cells or tissues. It is important to note that these products are not categorized as medicines or drugs, and they have not received approval from the FDA for the prevention, treatment, or cure of any medical condition, ailment, or disease. We must emphasize that any form of bodily introduction of these products into humans or animals is strictly prohibited by law. It is essential to adhere to these guidelines to ensure compliance with legal and ethical standards in research and experimentation.