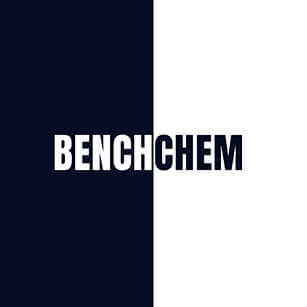
RIBONUCLEIC ACID, TRANSFER
- Click on QUICK INQUIRY to receive a quote from our team of experts.
- With the quality product at a COMPETITIVE price, you can focus more on your research.
Overview
Description
Transfer Ribonucleic Acid (tRNA) is a class of non-coding RNA essential for translating genetic information into proteins. It functions as an adaptor molecule, binding specific amino acids and delivering them to ribosomes during protein synthesis. tRNA is characterized by a cloverleaf secondary structure, which folds into an L-shaped tertiary conformation, enabling interactions with ribosomes and aminoacyl-tRNA synthetases . Key features include:
- Modified Nucleosides: tRNA contains post-transcriptional modifications such as thionucleosides (e.g., 4-thiouridine), dihydrouridine, and pseudouridine, which enhance stability and functionality .
- Amino Acid Specificity: Each tRNA is linked to a specific amino acid via aminoacyl-tRNA synthetases, ensuring fidelity in translation .
- Anticodon Loop: Recognizes mRNA codons through complementary base pairing .
Preparation Methods
Synthetic Routes and Reaction Conditions: The synthesis of transfer ribonucleic acid can be achieved through chemical synthesis and in vitro transcription. Chemical synthesis involves the stepwise addition of ribonucleotides to form the desired ribonucleic acid sequence. This method is suitable for producing short ribonucleic acid sequences. In vitro transcription, on the other hand, uses a DNA template and RNA polymerase to synthesize ribonucleic acid. This method is more efficient for producing longer ribonucleic acid sequences .
Industrial Production Methods: Large-scale production of transfer ribonucleic acid typically involves in vitro transcription due to its efficiency and scalability. The process includes the preparation of a DNA template, transcription using RNA polymerase, and purification of the resulting ribonucleic acid. High-performance liquid chromatography (HPLC) and liquid column chromatography are commonly used for purification .
Chemical Reactions Analysis
Methylthiolation: A Critical tRNA Modification
Methylthiolation involves adding a methyl sulfur (-SCH₃) group to tRNA, enhancing translational accuracy. The MiaB enzyme facilitates this process via a radical SAM (S-adenosylmethionine) mechanism in bacteria ( ):
Key Steps in Methylthiolation :
Step | Description | Catalytic Components |
---|---|---|
1 | Methyl group transfer from SAM to MiaB’s auxiliary Fe-S cluster | Radical SAM Fe-S cluster |
2 | Formation of a free radical via SAM cleavage | Fe-S cluster, SAM |
3 | Hydrogen abstraction from tRNA by the radical | Radical intermediate |
4 | Transfer of methylthio group to tRNA | Auxiliary Fe-S cluster |
-
Impact : Defective methylthiolation correlates with neuronal diseases, diabetes, and cancers due to translational errors .
-
Structural Insight : X-ray crystallography reveals tRNA geometry shifts to a tetrahedral conformation during methylthio group transfer .
Phosphoryl Transfer Reactions in tRNA Processing
Ribozymes catalyze phosphoryl transfer reactions critical for tRNA maturation. Examples include:
Ribozyme Types and Mechanisms ( ):
Ribozyme | Reaction Catalyzed | Catalytic Strategy |
---|---|---|
glmS | Self-cleavage via glucosamine-6-phosphate (GlcN6P) | GlcN6P acts as a general acid/base |
Hammerhead | RNA backbone cleavage | Metal ion stabilization, inline nucleophilic attack |
Twister | Site-specific cleavage | Base pairing and tertiary interactions |
-
Kinetics : glmS ribozyme achieves rates up to 5 s⁻¹ using GlcN6P as a cofactor .
-
Structural Basis : Twister ribozyme positions nucleobases (e.g., adenine) as acid/base catalysts within its catalytic pocket .
Hydrazine Reactivity with tRNA Modifications
Hydrazine selectively reacts with modified nucleosides, enabling detection and sequencing applications ( ):
Reactivity Profile of tRNA Modifications
Modification | Reactivity with Hydrazine (30%, 0°C) | Product Identified |
---|---|---|
Uridine (U) | 50–70% conversion to pyrazolone adduct | Hydrazine-U adduct |
Ψ (Pseudouridine) | No reaction | Intact Ψ |
m³C (3-methylcytidine) | >90% loss | Cytosine |
s⁴U (4-thiouridine) | Complete loss | Uracil-hydrazine adduct |
-
Applications : Enables discrimination of Ψ from U in sequencing and mass spectrometry (MS) workflows .
-
Mechanism : Hydrazine attacks electrophilic centers (e.g., C6 in U), forming stable adducts .
Enzymatic Removal of tRNA Modifications
The enzyme ALKBH1 demethylates tRNA, regulating translation efficiency ( ):
ALKBH1 Activity
Substrate | Modification Removed | Functional Consequence |
---|---|---|
tRNAᴬˡᵃ | m¹A (1-methyladenosine) | Stabilizes tRNA; enhances translation |
tRNAᴹᵉᵗ | m³C | Prevents tRNA degradation |
-
Biological Relevance : ALKBH1 knockout cells show reduced tRNA stability and impaired protein synthesis .
Mass Spectrometry (MS) for tRNA Analysis
Liquid chromatography-tandem MS (LC-MS/MS) maps tRNA modifications with high precision ( ):
Workflow for tRNA Modification Mapping
-
Digestion : tRNA → nucleosides (nuclease P1) or oligonucleotides (RNase A).
-
Chromatography : Separation via anion exchange or reverse-phase columns.
-
Detection : MS/MS identifies modifications (e.g., m⁷G, ac⁴C) based on mass shifts.
Example Data :
Modification | Mass Shift (Da) | Diagnostic Fragment Ions |
---|---|---|
m⁷G (7-methylguanosine) | +14.02 | m/z 298 → 152 |
ac⁴C (N⁴-acetylcytidine) | +42.01 | m/z 260 → 112 |
Aminoacylation: tRNA Charging Reactions
Aminoacyl-tRNA synthetases (ARS) catalyze tRNA charging via a two-step mechanism ( ):
Reaction Steps :
-
Adenylation : Amino acid + ATP → aminoacyl-AMP + PPᵢ.
-
Transfer : Aminoacyl-AMP + tRNA → aminoacyl-tRNA + AMP.
Scientific Research Applications
Gene Therapy
tRNA has been utilized in gene therapy approaches to correct genetic defects or enhance gene expression. For instance, research has demonstrated that engineered tRNA can restore function in cystic fibrosis patients by suppressing premature termination codons (PTCs) in the CFTR gene. This method has shown promise in preclinical studies, indicating its potential for treating genetic disorders associated with PTCs .
Cancer Treatment
Innovative strategies involving tRNA are being explored for cancer treatment. Specific tRNAs have been designed to target and inhibit the expression of oncogenes or to restore the function of tumor suppressor genes. For example, the use of tRNA to deliver therapeutic agents directly to cancer cells has been investigated, showing potential in enhancing the efficacy of existing treatments .
Biopharmaceutical Production
The production of biopharmaceuticals, including monoclonal antibodies and recombinant proteins, has benefited from advancements in tRNA technology. By optimizing tRNA availability and function within host cells, researchers can enhance protein yield and quality during recombinant protein production. This optimization is particularly relevant in the context of mammalian cell culture systems where post-translational modifications are critical .
Synthetic Biology
In synthetic biology, engineered tRNAs are being employed to expand the genetic code, allowing the incorporation of non-canonical amino acids into proteins. This capability opens new avenues for creating proteins with novel functionalities that could be used in therapeutics or industrial applications .
mRNA Stability and Translation Regulation
Recent studies have highlighted a novel regulatory mechanism where tRNAs influence mRNA stability and translation efficiency. The interaction between specific tRNAs and mRNAs can lead to mRNA decay or stabilization, affecting protein synthesis rates. This discovery sheds light on how cells manage protein production under various physiological conditions and could inform therapeutic strategies for diseases like cancer and metabolic disorders .
Case Studies
Mechanism of Action
Transfer ribonucleic acid exerts its effects by acting as an adaptor molecule during protein synthesis. It carries specific amino acids to the ribosome, where it pairs with the corresponding codon on the messenger ribonucleic acid through its anticodon loop. This pairing ensures the correct incorporation of amino acids into the growing polypeptide chain. The process is facilitated by aminoacyl-tRNA synthetases, which attach the appropriate amino acid to the transfer ribonucleic acid .
Comparison with Similar Compounds
Comparative Analysis of tRNA with Similar Compounds
tRNA vs. Messenger Ribonucleic Acid (mRNA)
Research Findings :
- tRNA modifications like 4-thiouridine protect against enzymatic degradation, unlike mRNA, which relies on 5' capping for stability .
- tRNA's anticodon loop directly interacts with mRNA codons, ensuring precise codon-anticodon pairing .
tRNA vs. Ribosomal Ribonucleic Acid (rRNA)
Research Findings :
- rRNA lacks the amino acid-binding capacity of tRNA but plays a structural role in ribosome assembly .
- tRNA's methyl groups (e.g., ribothymidine) are critical for synthetase recognition, a feature absent in rRNA .
tRNA vs. Viral Ribonucleic Acid (e.g., Tobacco Mosaic Virus RNA)
Research Findings :
- Viral RNA (e.g., Tobacco Mosaic Virus) shares nucleotide identity with yeast tRNA but lacks functional modifications like thionucleosides .
Research Findings :
- Yeast alanine tRNA's sequence complexity (e.g., large oligonucleotide fragments) contrasts with synthetic oligonucleotides designed for simplicity .
Functional and Structural Insights from Research
- Methylation and Tertiary Structure : Methylation of tRNA (e.g., at the wobble position) stabilizes its L-shaped structure and ensures proper codon recognition .
- Thionucleosides in tRNA: 4-Thiouridine in Bacillus subtilis tRNA enhances UV resistance and amino acid acceptance, a feature critical for bacterial survival .
- Conformational Flexibility : Tyrosine-specific tRNAs exhibit early melting transitions, indicating structural adaptability during translation .
Data Table: Key Modifications in tRNA vs. Other RNAs
Modification Type | tRNA | mRNA | rRNA |
---|---|---|---|
Thionucleosides | Present (e.g., 4-thiouridine) | Absent | Absent |
Dihydrouridine | Abundant | Absent | Rare |
Pseudouridine | Common | Absent | Present in some regions |
Methylation | Extensive | 5' cap only | Limited |
Functional Impact | Stability, synthetase binding | Stability, export | Ribosome assembly |
References |
Q & A
Basic Research Questions
Q. What are the best practices for isolating high-purity tRNA from eukaryotic cells?
Methodological Answer:
- Source Material Preparation: Use fresh or flash-frozen eukaryotic cells (e.g., S. cerevisiae baker’s yeast) to minimize RNase degradation .
- Extraction Protocol: Employ the phenol-chloroform method for intact tRNA recovery. For plant or animal tissues, combine with SDS to dissociate ribonucleoproteins .
- DNase Treatment: After ethanol precipitation, treat samples with RNase-free DNase I to remove contaminating genomic DNA .
- Quality Control: Verify purity via A260/A280 ratios (1.8–2.0) and agarose gel electrophoresis to confirm intact tRNA bands .
Table 1: Comparison of tRNA Isolation Methods
Method | Yield (µg/g tissue) | Purity (A260/A280) | Key Applications |
---|---|---|---|
Phenol-Chloroform | 50–100 | 1.9–2.0 | Structural studies |
Phenol-SDS | 30–60 | 1.8–1.9 | Quantitative recovery |
Commercial Kits | 20–50 | 1.7–1.9 | High-throughput workflows |
Q. How can researchers ensure tRNA integrity during extraction and storage?
Methodological Answer:
- RNase Inhibition: Use RNase inhibitors (e.g., DEPC-treated water) and work in a laminar flow hood to prevent contamination .
- Storage Conditions: Dissolve tRNA in RNase-free TE buffer (pH 7.0) and store at –80°C in single-use aliquots to avoid freeze-thaw cycles .
- Integrity Checks: Perform capillary electrophoresis (e.g., Bioanalyzer) to assess tRNA size distribution and detect degradation .
Q. What methodologies are used to quantify tRNA concentration and assess purity?
Methodological Answer:
- UV Spectrophotometry: Measure A260/A280 ratios; values <1.8 indicate protein contamination .
- Fluorometric Assays: Use RNA-specific dyes (e.g., RiboGreen) for enhanced sensitivity in low-concentration samples .
- Agarose Gel Electrophoresis: Visualize sharp tRNA bands (~70–90 nucleotides) to confirm absence of smearing .
Advanced Research Questions
Q. How can researchers resolve discrepancies in tRNA modification profiles across sequencing platforms?
Methodological Answer:
- Platform-Specific Calibration: Use reference tRNA sequences with known modifications (e.g., from S. cerevisiae) to calibrate RNA-Seq or mass spectrometry data .
- Comparative Analysis of RNA Digests (CARD): Label reference tRNAs with ¹⁶O and candidate tRNAs with ¹⁸O for mass spectrometry-based sequencing to identify modifications .
- Cross-Validation: Combine microarray analysis with Northern blotting to confirm modification sites .
Table 2: Advantages and Limitations of tRNA Sequencing Methods
Method | Sensitivity | Modification Detection | Throughput |
---|---|---|---|
RNA-Seq | High | Limited | High |
Mass Spectrometry | Moderate | High | Low |
CARD Approach | High | High | Moderate |
Q. What experimental design considerations are critical for studying tRNA-export complexes (e.g., Exportin-T interactions) in vitro?
Methodological Answer:
- Reconstitution Assays: Purify Exportin-T and tRNA using affinity chromatography (e.g., His-tagged proteins) and validate binding via gel-shift assays .
- Competition Controls: Include unlabeled tRNA to confirm specificity of radioactive or fluorescently labeled tRNA interactions .
- Buffer Optimization: Use transport buffer (pH 7.4) with RanGTP to mimic nuclear export conditions .
- Ethical Compliance: Follow NIH guidelines for recombinant DNA handling and disclose conflicts of interest per institutional protocols .
Q. What strategies improve detection of low-abundance tRNA variants in heterogeneous samples?
Methodological Answer:
- Enrichment Techniques: Use biotinylated probes complementary to target tRNA variants for pull-down assays .
- Amplification-Free Sequencing: Apply direct RNA sequencing (Oxford Nanopore) to avoid PCR bias .
- Statistical Modeling: Implement machine learning algorithms to distinguish signal from noise in high-throughput datasets .
Q. How can researchers address conflicting data on tRNA charging efficiency in amino acid starvation studies?
Methodological Answer:
- Standardized Controls: Include internal standards (e.g., synthetic charged tRNA) in aminoacylation assays .
- Time-Course Experiments: Monitor charging kinetics at multiple time points to capture dynamic changes .
- Meta-Analysis: Aggregate data from public repositories (e.g., GEO) to identify consensus patterns .
Properties
CAS No. |
120991-82-4 |
---|---|
Molecular Formula |
C11H10BrNO |
Molecular Weight |
0 |
Origin of Product |
United States |
Disclaimer and Information on In-Vitro Research Products
Please be aware that all articles and product information presented on BenchChem are intended solely for informational purposes. The products available for purchase on BenchChem are specifically designed for in-vitro studies, which are conducted outside of living organisms. In-vitro studies, derived from the Latin term "in glass," involve experiments performed in controlled laboratory settings using cells or tissues. It is important to note that these products are not categorized as medicines or drugs, and they have not received approval from the FDA for the prevention, treatment, or cure of any medical condition, ailment, or disease. We must emphasize that any form of bodily introduction of these products into humans or animals is strictly prohibited by law. It is essential to adhere to these guidelines to ensure compliance with legal and ethical standards in research and experimentation.