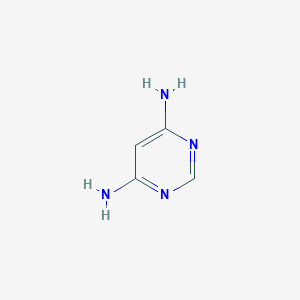
4,6-Diaminopyrimidine
Overview
Description
4,6-Diaminopyrimidine (DAP) is a class of organic chemical compounds that include two amine groups on a pyrimidine ring . It is used in the preparation of pharmaceutical goods .
Synthesis Analysis
A series of substituted diheteroaryl sulfides was synthesized by the reaction of 4,6-diaminopyrimidine-2(1H)-thione with fluoro- and chloroheteroaromatic compounds in the presence of Na2CO3 in acetonitrile under reflux conditions . The structures of the synthesized compounds were confirmed by IR, 1H, 19F, and 13C NMR spectroscopy, as well as elemental analysis and X-ray structural analysis .
Molecular Structure Analysis
The molecular formula of 4,6-Diaminopyrimidine is C4H6N4, with an average mass of 110.117 Da and a monoisotopic mass of 110.059242 Da .
Chemical Reactions Analysis
4,6-Diaminopyrimidine-2(1H)-thione reacts with fluoro- and chloroheteroaromatic compounds chemoselectively as S- rather than N-nucleophile . The structures of the synthesized compounds were confirmed by IR, 1H, 19F, and 13C NMR spectroscopy, as well as elemental analysis and X-ray structural analysis .
Physical And Chemical Properties Analysis
4,6-Diaminopyrimidine has a molecular weight of 110.12 and is a solid at room temperature . It should be stored in a dark place, sealed in dry, at room temperature .
Scientific Research Applications
Pharmaceuticals: Anticancer Agents
4,6-Diaminopyrimidine serves as a core structure in the synthesis of compounds with potent anticancer activities. Its derivatives have been explored for their ability to inhibit cell proliferation in various cancer cell lines. The pyrimidine ring, being a key component of DNA and RNA, allows for the design of molecules that can interfere with DNA replication and transcription processes, making it a valuable target in cancer therapy .
Antimicrobial and Antifungal Applications
This compound has shown promise in the development of new antimicrobial and antifungal agents. Its structural similarity to natural nucleotides enables it to act as a competitive inhibitor for enzymes involved in nucleotide synthesis, which is crucial for the growth and multiplication of pathogenic microorganisms .
Agricultural Chemistry: Pesticides
In the field of agricultural chemistry, 4,6-Diaminopyrimidine derivatives are used to create pesticides. These compounds can disrupt the life cycle of pests at a molecular level, providing an effective means of protecting crops without harming the environment .
Material Science: Organic Semiconductors
Researchers are investigating the use of 4,6-Diaminopyrimidine in the synthesis of organic semiconductors. Its ability to donate electrons makes it a candidate for creating materials that can be used in organic light-emitting diodes (OLEDs) and other electronic devices .
Biochemistry: Enzyme Inhibitors
Due to its structural properties, 4,6-Diaminopyrimidine is a potential candidate for the design of enzyme inhibitors. It can mimic the transition state of enzymatic reactions or bind to the active site of enzymes, thereby inhibiting their function, which is a common strategy in drug development .
Neuroscience: Neuroprotective Agents
The compound’s derivatives are being explored for their neuroprotective properties. They may offer therapeutic potential in neurodegenerative diseases by protecting neuronal cells from damage or death .
Cosmetics: Hair Loss Treatments
4,6-Diaminopyrimidine is found in hair loss treatment formulations. It is known to reduce hair shedding and increase hair mass and density, making it a valuable ingredient in cosmetic products aimed at treating hair disorders .
Analytical Chemistry: Chromatography Standards
In analytical chemistry, 4,6-Diaminopyrimidine can be used as a standard in chromatography to help identify and quantify substances within a sample. Its well-defined properties make it suitable for use as a reference compound in various analytical techniques .
Mechanism of Action
Target of Action
4,6-Diaminopyrimidine is a structural analog of pyrimidine, a key component of DNA and RNA. It has been found to target Hematopoietic Progenitor Kinase 1 (HPK1) , a member of the germinal center kinase family of serine/threonine kinases . HPK1 is mainly expressed by hematopoietic cells and plays a crucial role in immune response .
Mode of Action
It is known that pyrimidine analogs like pyrimethamine inhibit the dihydrofolate reductase of plasmodia, thereby blocking the biosynthesis of purines and pyrimidines, which are essential for dna synthesis and cell multiplication . This leads to failure of nuclear division at the time of schizont formation in erythrocytes and liver .
Biochemical Pathways
It is known that pyrimidine analogs like pyrimethamine inhibit the dihydrofolate reductase, thereby blocking the biosynthesis of purines and pyrimidines . This inhibition disrupts DNA synthesis and cell multiplication, leading to the death of the parasite .
Pharmacokinetics
A study on a similar compound, 14-o-[(4,6-diaminopyrimidine-2-yl)thioacetyl] mutilin (dptm), showed that after intravenous, intramuscular, and oral administrations, dptm was rapidly absorbed and metabolized in rats . The half-life of DPTM was found to be between 1.70–4.70 hours for different doses .
Result of Action
It is known that pyrimidine analogs like pyrimethamine lead to the failure of nuclear division at the time of schizont formation in erythrocytes and liver, resulting in the death of the parasite .
Safety and Hazards
Future Directions
properties
IUPAC Name |
pyrimidine-4,6-diamine | |
---|---|---|
Source | PubChem | |
URL | https://pubchem.ncbi.nlm.nih.gov | |
Description | Data deposited in or computed by PubChem | |
InChI |
InChI=1S/C4H6N4/c5-3-1-4(6)8-2-7-3/h1-2H,(H4,5,6,7,8) | |
Source | PubChem | |
URL | https://pubchem.ncbi.nlm.nih.gov | |
Description | Data deposited in or computed by PubChem | |
InChI Key |
MISVBCMQSJUHMH-UHFFFAOYSA-N | |
Source | PubChem | |
URL | https://pubchem.ncbi.nlm.nih.gov | |
Description | Data deposited in or computed by PubChem | |
Canonical SMILES |
C1=C(N=CN=C1N)N | |
Source | PubChem | |
URL | https://pubchem.ncbi.nlm.nih.gov | |
Description | Data deposited in or computed by PubChem | |
Molecular Formula |
C4H6N4 | |
Source | PubChem | |
URL | https://pubchem.ncbi.nlm.nih.gov | |
Description | Data deposited in or computed by PubChem | |
DSSTOX Substance ID |
DTXSID00275776 | |
Record name | 4,6-Diaminopyrimidine | |
Source | EPA DSSTox | |
URL | https://comptox.epa.gov/dashboard/DTXSID00275776 | |
Description | DSSTox provides a high quality public chemistry resource for supporting improved predictive toxicology. | |
Molecular Weight |
110.12 g/mol | |
Source | PubChem | |
URL | https://pubchem.ncbi.nlm.nih.gov | |
Description | Data deposited in or computed by PubChem | |
Product Name |
4,6-Diaminopyrimidine | |
CAS RN |
2434-56-2 | |
Record name | 4,6-Pyrimidinediamine | |
Source | DTP/NCI | |
URL | https://dtp.cancer.gov/dtpstandard/servlet/dwindex?searchtype=NSC&outputformat=html&searchlist=211429 | |
Description | The NCI Development Therapeutics Program (DTP) provides services and resources to the academic and private-sector research communities worldwide to facilitate the discovery and development of new cancer therapeutic agents. | |
Explanation | Unless otherwise indicated, all text within NCI products is free of copyright and may be reused without our permission. Credit the National Cancer Institute as the source. | |
Record name | 4,6-Diaminopyrimidine | |
Source | EPA DSSTox | |
URL | https://comptox.epa.gov/dashboard/DTXSID00275776 | |
Description | DSSTox provides a high quality public chemistry resource for supporting improved predictive toxicology. | |
Record name | 4,6-DIAMINOPYRIMIDINE | |
Source | European Chemicals Agency (ECHA) | |
URL | https://echa.europa.eu/information-on-chemicals | |
Description | The European Chemicals Agency (ECHA) is an agency of the European Union which is the driving force among regulatory authorities in implementing the EU's groundbreaking chemicals legislation for the benefit of human health and the environment as well as for innovation and competitiveness. | |
Explanation | Use of the information, documents and data from the ECHA website is subject to the terms and conditions of this Legal Notice, and subject to other binding limitations provided for under applicable law, the information, documents and data made available on the ECHA website may be reproduced, distributed and/or used, totally or in part, for non-commercial purposes provided that ECHA is acknowledged as the source: "Source: European Chemicals Agency, http://echa.europa.eu/". Such acknowledgement must be included in each copy of the material. ECHA permits and encourages organisations and individuals to create links to the ECHA website under the following cumulative conditions: Links can only be made to webpages that provide a link to the Legal Notice page. | |
Record name | 4,6-Pyrimidinediamine | |
Source | FDA Global Substance Registration System (GSRS) | |
URL | https://gsrs.ncats.nih.gov/ginas/app/beta/substances/RD899M5PW6 | |
Description | The FDA Global Substance Registration System (GSRS) enables the efficient and accurate exchange of information on what substances are in regulated products. Instead of relying on names, which vary across regulatory domains, countries, and regions, the GSRS knowledge base makes it possible for substances to be defined by standardized, scientific descriptions. | |
Explanation | Unless otherwise noted, the contents of the FDA website (www.fda.gov), both text and graphics, are not copyrighted. They are in the public domain and may be republished, reprinted and otherwise used freely by anyone without the need to obtain permission from FDA. Credit to the U.S. Food and Drug Administration as the source is appreciated but not required. | |
Retrosynthesis Analysis
AI-Powered Synthesis Planning: Our tool employs the Template_relevance Pistachio, Template_relevance Bkms_metabolic, Template_relevance Pistachio_ringbreaker, Template_relevance Reaxys, Template_relevance Reaxys_biocatalysis model, leveraging a vast database of chemical reactions to predict feasible synthetic routes.
One-Step Synthesis Focus: Specifically designed for one-step synthesis, it provides concise and direct routes for your target compounds, streamlining the synthesis process.
Accurate Predictions: Utilizing the extensive PISTACHIO, BKMS_METABOLIC, PISTACHIO_RINGBREAKER, REAXYS, REAXYS_BIOCATALYSIS database, our tool offers high-accuracy predictions, reflecting the latest in chemical research and data.
Strategy Settings
Precursor scoring | Relevance Heuristic |
---|---|
Min. plausibility | 0.01 |
Model | Template_relevance |
Template Set | Pistachio/Bkms_metabolic/Pistachio_ringbreaker/Reaxys/Reaxys_biocatalysis |
Top-N result to add to graph | 6 |
Feasible Synthetic Routes
Disclaimer and Information on In-Vitro Research Products
Please be aware that all articles and product information presented on BenchChem are intended solely for informational purposes. The products available for purchase on BenchChem are specifically designed for in-vitro studies, which are conducted outside of living organisms. In-vitro studies, derived from the Latin term "in glass," involve experiments performed in controlled laboratory settings using cells or tissues. It is important to note that these products are not categorized as medicines or drugs, and they have not received approval from the FDA for the prevention, treatment, or cure of any medical condition, ailment, or disease. We must emphasize that any form of bodily introduction of these products into humans or animals is strictly prohibited by law. It is essential to adhere to these guidelines to ensure compliance with legal and ethical standards in research and experimentation.