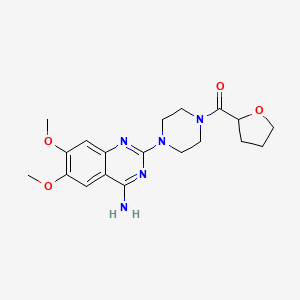
(R)-Terazosin
Description
Terazosin is a member of quinazolines, a member of piperazines, a member of furans and a primary amino compound. It has a role as an antineoplastic agent, an antihypertensive agent and an alpha-adrenergic antagonist.
Terazosin is a quinazoline derivative alpha-1-selective adrenergic blocking agent indicated for benign prostatic hyperplasia and hypertension. Terazosin blocks adrenaline's action on alpha-1 adrenergic receptors, causing relaxation of smooth muscle in blood vessels and the prostate.
Terazosin is a nonselective alpha-1 adrenergic antagonist used in the therapy of hypertension and benign prostatic hypertrophy. Terazosin therapy is associated with a low rate of transient serum aminotransferase elevations and to rare instances of clinically apparent acute liver injury.
Properties
IUPAC Name |
[4-(4-amino-6,7-dimethoxyquinazolin-2-yl)piperazin-1-yl]-[(2R)-oxolan-2-yl]methanone | |
---|---|---|
Source | PubChem | |
URL | https://pubchem.ncbi.nlm.nih.gov | |
Description | Data deposited in or computed by PubChem | |
InChI |
InChI=1S/C19H25N5O4/c1-26-15-10-12-13(11-16(15)27-2)21-19(22-17(12)20)24-7-5-23(6-8-24)18(25)14-4-3-9-28-14/h10-11,14H,3-9H2,1-2H3,(H2,20,21,22)/t14-/m1/s1 | |
Source | PubChem | |
URL | https://pubchem.ncbi.nlm.nih.gov | |
Description | Data deposited in or computed by PubChem | |
InChI Key |
VCKUSRYTPJJLNI-CQSZACIVSA-N | |
Source | PubChem | |
URL | https://pubchem.ncbi.nlm.nih.gov | |
Description | Data deposited in or computed by PubChem | |
Canonical SMILES |
COC1=C(C=C2C(=C1)C(=NC(=N2)N3CCN(CC3)C(=O)C4CCCO4)N)OC | |
Source | PubChem | |
URL | https://pubchem.ncbi.nlm.nih.gov | |
Description | Data deposited in or computed by PubChem | |
Isomeric SMILES |
COC1=C(C=C2C(=C1)C(=NC(=N2)N3CCN(CC3)C(=O)[C@H]4CCCO4)N)OC | |
Source | PubChem | |
URL | https://pubchem.ncbi.nlm.nih.gov | |
Description | Data deposited in or computed by PubChem | |
Molecular Formula |
C19H25N5O4 | |
Source | PubChem | |
URL | https://pubchem.ncbi.nlm.nih.gov | |
Description | Data deposited in or computed by PubChem | |
Molecular Weight |
387.4 g/mol | |
Source | PubChem | |
URL | https://pubchem.ncbi.nlm.nih.gov | |
Description | Data deposited in or computed by PubChem | |
Retrosynthesis Analysis
AI-Powered Synthesis Planning: Our tool employs the Template_relevance Pistachio, Template_relevance Bkms_metabolic, Template_relevance Pistachio_ringbreaker, Template_relevance Reaxys, Template_relevance Reaxys_biocatalysis model, leveraging a vast database of chemical reactions to predict feasible synthetic routes.
One-Step Synthesis Focus: Specifically designed for one-step synthesis, it provides concise and direct routes for your target compounds, streamlining the synthesis process.
Accurate Predictions: Utilizing the extensive PISTACHIO, BKMS_METABOLIC, PISTACHIO_RINGBREAKER, REAXYS, REAXYS_BIOCATALYSIS database, our tool offers high-accuracy predictions, reflecting the latest in chemical research and data.
Strategy Settings
Precursor scoring | Relevance Heuristic |
---|---|
Min. plausibility | 0.01 |
Model | Template_relevance |
Template Set | Pistachio/Bkms_metabolic/Pistachio_ringbreaker/Reaxys/Reaxys_biocatalysis |
Top-N result to add to graph | 6 |
Feasible Synthetic Routes
Q1: How does (R)-terazosin interact with α1-adrenoceptors compared to its enantiomer (S)-terazosin?
A1: Research suggests that both this compound and (S)-terazosin exhibit similar binding affinities for α1-adrenoceptor subtypes. Studies using cloned human α1-adrenoceptors expressed in cell lines found no significant difference in binding affinities between the two enantiomers for the α1a, α1b, and α1d subtypes [, ]. This indicates that the stereochemistry at the tetrahydrofuran moiety does not significantly influence the interaction with these receptor subtypes.
Q2: Does the stereochemistry of terazosin impact its pharmacokinetic profile?
A2: Interestingly, despite similar receptor binding affinities, this compound demonstrates different pharmacokinetics compared to (S)-terazosin. A study in healthy Chinese male subjects revealed that after a single oral dose of racemic terazosin, the plasma concentrations of this compound were consistently higher than those of (S)-terazosin []. The area under the plasma concentration-time curve (AUC0-∞) and maximum plasma concentration (Cmax) were significantly greater for this compound, suggesting stereoselective pharmacokinetics in humans [].
Q3: Does this compound exhibit selectivity for α1-adrenoceptors over α2-adrenoceptors?
A3: While both enantiomers of terazosin display high affinity for α1-adrenoceptors, this compound exhibits a lower affinity for α2b-adrenoceptors compared to (S)-terazosin and racemic terazosin []. This difference in binding affinity suggests a slightly higher selectivity of this compound for α1-adrenoceptors over the α2b subtype, potentially leading to a different side effect profile compared to the racemate.
Q4: Are there any studies investigating the use of radiolabeled this compound as a tool for studying α1-adrenoceptors?
A4: Yes, research has explored the use of [3H]R-terazosin as a radioligand for studying α1-adrenoceptors []. Studies utilizing [3H]R-terazosin in rat neonatal lung membrane preparations, which contain both α1- and α2-adrenoceptors, revealed selective binding to a single site consistent with α1-adrenoceptors []. This suggests that [3H]R-terazosin may be a valuable tool for investigating α1-adrenoceptor function in tissues expressing multiple adrenoceptor subtypes.
Disclaimer and Information on In-Vitro Research Products
Please be aware that all articles and product information presented on BenchChem are intended solely for informational purposes. The products available for purchase on BenchChem are specifically designed for in-vitro studies, which are conducted outside of living organisms. In-vitro studies, derived from the Latin term "in glass," involve experiments performed in controlled laboratory settings using cells or tissues. It is important to note that these products are not categorized as medicines or drugs, and they have not received approval from the FDA for the prevention, treatment, or cure of any medical condition, ailment, or disease. We must emphasize that any form of bodily introduction of these products into humans or animals is strictly prohibited by law. It is essential to adhere to these guidelines to ensure compliance with legal and ethical standards in research and experimentation.