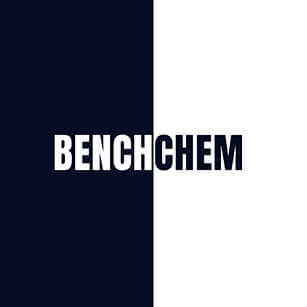
Xylan
- Click on QUICK INQUIRY to receive a quote from our team of experts.
- With the quality product at a COMPETITIVE price, you can focus more on your research.
Overview
Description
Xylan is a type of hemicellulose, a polysaccharide consisting mainly of xylose residues. It is found in plants, particularly in the secondary cell walls of dicots and all cell walls of grasses. This compound is the third most abundant biopolymer on Earth, after cellulose and chitin . It plays a crucial role in the integrity of the plant cell wall and contributes to plant growth and development .
Mechanism of Action
Target of Action
Xylan, the second most abundant naturally occurring renewable polysaccharide on earth , is a complex heteropolysaccharide found in plant cell walls . The primary targets of this compound are the β-1,4-glycoside linkages in the this compound backbone . These linkages are cleaved by xylanases, a group of enzymes that include endo -1,4-β-D-xylanases (EC 3.2.1.8), β-D-xylosidases (E.C. 3.2.1.37), α-L-arabinofuranosidases (E.C. 3.2.1.55), acetylthis compound esterase (EC 3.1.1.72), and α-glucuronidase (EC 3.2.1.139) .
Mode of Action
Xylanases interact with their targets by cleaving the heterogeneous β-1,4-glycoside linkage . This interaction results in the depolymerization of this compound into simple monosaccharides and xylooligosaccharides . The catalytic site of xylanases contains two glutamate residues, one acting as a nucleophile and the other as an acid/base catalyst .
Biochemical Pathways
The breakdown of this compound involves a variety of enzymes, including main- and side-chain acting enzymes . Xylanase is a main-chain enzyme that randomly cleaves the β-1,4 linkages between the xylopyranosyl residues in the this compound backbone . This enzyme presents varying folds, mechanisms of action, substrate specificities, hydrolytic activities, and physicochemical characteristics .
Pharmacokinetics
It’s known that xylanases are genetically single chain glycoproteins, active between ph 45–65 and temperature 40–60 °C .
Result of Action
The action of xylanases results in the breakdown of the complex structure of this compound into its constituent sugars . This process requires the synergistic action of various enzymes, each targeting appropriate bonds of this compound . The breakdown of this compound by xylanases is essential for the efficient utilization of lignocellulosic resources .
Action Environment
The action of xylanases is influenced by environmental factors such as pH and temperature . These enzymes are primarily produced by a variety of microorganisms, including fungi, algae, yeast, and bacteria . The efficiency of this compound degradation can be influenced by the sequential secretion and different action modes of xylanases .
Biochemical Analysis
Biochemical Properties
Xylan plays a significant role in biochemical reactions, particularly in the degradation and utilization of plant biomass. It interacts with various enzymes, including xylanases, which are responsible for breaking down the β-1,4-glycosidic linkages in this compound. Xylanases are produced by a wide range of microorganisms, including bacteria, fungi, yeast, and algae . These enzymes include endo-1,4-β-D-xylanases, β-D-xylosidases, α-glucuronidases, acetylthis compound esterases, α-L-arabinofuranosidases, p-coumaric esterases, and ferulic acid esterases . The interactions between this compound and these enzymes are crucial for the depolymerization of this compound into simple monosaccharides and xylooligosaccharides, which can be further utilized in various industrial applications.
Cellular Effects
This compound influences various cellular processes, particularly in plant cells. It is essential for the normal deposition and assembly of secondary cell walls, contributing to the structural integrity and rigidity of the cell wall . This compound also affects cell signaling pathways, gene expression, and cellular metabolism. For example, genetic analyses in Arabidopsis have shown that this compound is indispensable for the proper formation of secondary cell walls . Additionally, this compound’s interactions with other cell wall components, such as cellulose and lignin, play a critical role in maintaining cell wall structure and function.
Molecular Mechanism
The molecular mechanism of this compound’s action involves its interactions with various biomolecules, including enzymes and proteins. This compound synthases, glucuronyltransferases, and methyltransferases are involved in the biosynthesis and modification of this compound . These enzymes catalyze the elongation of the this compound backbone and the addition of various substituents, such as glucuronic acid and arabinose . The binding interactions between this compound and these enzymes are crucial for determining the structure and function of this compound in the cell wall. Additionally, this compound’s interactions with xylanases and other degrading enzymes are essential for its depolymerization and utilization in various biochemical processes .
Temporal Effects in Laboratory Settings
In laboratory settings, the effects of this compound can change over time due to its stability, degradation, and long-term effects on cellular function. This compound is relatively stable under normal conditions, but it can be degraded by xylanases and other degrading enzymes . The stability and degradation of this compound can influence its long-term effects on cellular function, particularly in in vitro and in vivo studies. For example, the degradation of this compound by xylanases can lead to the release of simple monosaccharides and xylooligosaccharides, which can be further utilized in various biochemical processes .
Dosage Effects in Animal Models
The effects of this compound can vary with different dosages in animal models. At low doses, this compound may have minimal effects on cellular function, while at higher doses, it may exhibit toxic or adverse effects. For example, high doses of this compound may lead to the accumulation of degradation products, which can interfere with normal cellular processes . Additionally, threshold effects may be observed, where a certain dosage of this compound is required to elicit a specific biochemical response.
Metabolic Pathways
This compound is involved in various metabolic pathways, particularly in the degradation and utilization of plant biomass. It interacts with enzymes such as xylanases, which catalyze the depolymerization of this compound into simple monosaccharides and xylooligosaccharides . These degradation products can be further metabolized through various biochemical pathways, contributing to the overall metabolic flux and metabolite levels in the cell.
Transport and Distribution
This compound is transported and distributed within cells and tissues through various mechanisms. It interacts with transporters and binding proteins that facilitate its movement and localization within the cell . For example, this compound is deposited together with cellulose in the secondary cell walls of xylem cells in both rice and Arabidopsis . The transport and distribution of this compound are crucial for its proper function and activity within the cell.
Subcellular Localization
This compound is localized in specific subcellular compartments, particularly in the cell wall. It is deposited together with cellulose in the secondary cell walls of xylem cells, where it plays a crucial role in maintaining structural integrity . The subcellular localization of this compound is influenced by various targeting signals and post-translational modifications that direct it to specific compartments or organelles within the cell.
Preparation Methods
Synthetic Routes and Reaction Conditions: Xylan can be extracted from lignocellulosic biomass using various methods. The extraction process typically involves the use of alkaline solutions such as sodium hydroxide or potassium hydroxide. Sodium hydroxide is often preferred due to its higher yield . The extracted this compound is then purified through processes such as precipitation, dialysis, and drying .
Industrial Production Methods: In industrial settings, this compound is primarily produced from agricultural residues and hardwoods. The process involves the mechanical and chemical treatment of biomass to extract this compound. The extracted this compound is then purified and processed into various forms for different applications .
Chemical Reactions Analysis
Types of Reactions: Xylan undergoes various chemical reactions, including hydrolysis, oxidation, and esterification.
Common Reagents and Conditions:
Hydrolysis: this compound can be hydrolyzed using acids such as sulfuric acid or enzymes like xylanases.
Oxidation: this compound can be oxidized using oxidizing agents such as hydrogen peroxide or potassium permanganate.
Esterification: this compound can be esterified using reagents like acetic anhydride or succinic anhydride to produce this compound esters.
Major Products: The major products formed from these reactions include xylose, xylooligosaccharides, and various this compound derivatives such as this compound esters and ethers .
Scientific Research Applications
Xylan has a wide range of applications in various fields:
Chemistry: this compound is used as a raw material for the production of biofuels and biochemicals.
Biology: this compound and its derivatives are used in the study of plant cell wall structure and function.
Industry: this compound is used in the paper and pulp industry for biobleaching and improving paper quality.
Comparison with Similar Compounds
Xylan is similar to other hemicelluloses such as glucuronothis compound, arabinothis compound, and glucomannan. it is unique in its structure and composition:
Glucuronothis compound: Contains glucuronic acid residues and is found in hardwoods.
Arabinothis compound: Contains arabinose residues and is found in cereals and grasses.
Glucomannan: Contains mannose and glucose residues and is found in softwoods.
This compound’s unique structure and properties make it suitable for a wide range of applications, from biofuel production to biomedical applications .
Biological Activity
Xylan is a significant hemicellulose component found in the cell walls of plants, primarily contributing to structural integrity and interaction with other polysaccharides like cellulose and lignin. This article delves into the biological activity of this compound, examining its biosynthesis, structural characteristics, and various biological effects, including immunomodulatory, antioxidant, and anticoagulant activities.
Structure and Biosynthesis of this compound
This compound is composed of a backbone of xylose residues that can be modified with various substituents, including acetyl groups and arabinose side chains. The structure of this compound varies across different plant species and tissues, influencing its functional properties within the cell wall.
Key Features of this compound Structure
- Backbone Composition : Primarily consists of β-1,4-linked xylose units.
- Conformational States : this compound can adopt two helical conformations: a twofold screw conformation for interaction with cellulose and a threefold screw conformation that facilitates its role in the cell wall matrix.
- Modification Patterns : The presence of side chains affects the interaction between this compound, cellulose, and lignin, crucial for maintaining cell wall stability.
Biosynthetic Pathway
This compound biosynthesis occurs in the Golgi apparatus through the action of glycosyltransferases. Key enzymes involved include:
- Irregular Xylem 10 (IRX10) : Responsible for extending the this compound backbone.
- This compound Synthase Complex (XSC) : Comprises multiple glycosyltransferases that assist in synthesizing this compound in vivo.
Biological Activities of this compound
Research has highlighted several biological activities associated with this compound:
1. Immunomodulatory Activity
Studies have shown that this compound can stimulate cell proliferation and enhance the production of anti-inflammatory cytokines. For instance, this compound derived from Dinizia excelsa demonstrated significant immunomodulatory effects in vitro, indicating its potential as an immune system enhancer .
2. Antioxidant Activity
While this compound exhibits some antioxidant properties, its effectiveness is generally lower than that of well-known antioxidants such as ascorbic acid and butylated hydroxytoluene (BHT). This suggests that while this compound contributes to oxidative stress reduction, it may not be sufficient as a standalone antioxidant agent .
3. Anticoagulant Activity
This compound has been evaluated for its anticoagulant properties. Although it shows some activity, it is significantly lower compared to heparin, indicating that while it may have potential applications in blood coagulation modulation, further research is necessary to enhance its efficacy .
Case Study 1: this compound's Role in Plant Growth
Research involving this compound-deficient mutants has revealed critical insights into its role in plant development. These mutants typically exhibit stunted growth and deformed vascular tissues due to compromised cell wall integrity. This underscores the importance of this compound in maintaining structural function within plant cells .
Case Study 2: Applications in Biotechnology
This compound's unique properties have made it a subject of interest for various biotechnological applications. For instance, enzymatic degradation of this compound is utilized in biofuel production processes where lignocellulosic biomass is converted into fermentable sugars .
Summary Table of Biological Activities
Biological Activity | Description | Comparison |
---|---|---|
Immunomodulatory | Stimulates cell proliferation and anti-inflammatory cytokine production | Effective but requires further exploration |
Antioxidant | Reduces oxidative stress | Weaker than ascorbic acid/BHT |
Anticoagulant | Low anticoagulant activity compared to heparin | Potential for therapeutic applications |
Properties
CAS No. |
9014-63-5 |
---|---|
Molecular Formula |
(C5H8O4)n.; (C5H7O4R)n |
Appearance |
White to Off-White Solid |
melting_point |
>180°C (dec.) |
Purity |
98% |
solubility |
Water (Slightly) |
storage |
Room Temperature |
Synonyms |
POLY[BETA-D-XYLOPYRANOSE(1->4)]; XYLAN; XYLAN EX BEECHWOOD; XYLAN, OAT SPELTS; XYLAN OATS SPELT; (1,4-beta-D-Xylan)n; (1,4-beta-D-Xylan)n+1; 1,4-beta-D-Xylan |
Origin of Product |
United States |
Disclaimer and Information on In-Vitro Research Products
Please be aware that all articles and product information presented on BenchChem are intended solely for informational purposes. The products available for purchase on BenchChem are specifically designed for in-vitro studies, which are conducted outside of living organisms. In-vitro studies, derived from the Latin term "in glass," involve experiments performed in controlled laboratory settings using cells or tissues. It is important to note that these products are not categorized as medicines or drugs, and they have not received approval from the FDA for the prevention, treatment, or cure of any medical condition, ailment, or disease. We must emphasize that any form of bodily introduction of these products into humans or animals is strictly prohibited by law. It is essential to adhere to these guidelines to ensure compliance with legal and ethical standards in research and experimentation.