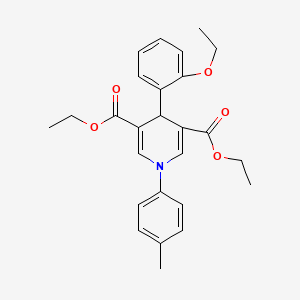
Diethyl 4-(2-ethoxyphenyl)-1-(4-methylphenyl)-1,4-dihydropyridine-3,5-dicarboxylate
- Click on QUICK INQUIRY to receive a quote from our team of experts.
- With the quality product at a COMPETITIVE price, you can focus more on your research.
Overview
Description
3,5-DIETHYL 4-(2-ETHOXYPHENYL)-1-(4-METHYLPHENYL)-1,4-DIHYDROPYRIDINE-3,5-DICARBOXYLATE is a complex organic compound belonging to the dihydropyridine class This compound is characterized by its unique structure, which includes ethyl, ethoxyphenyl, and methylphenyl groups attached to a dihydropyridine core
Preparation Methods
Synthetic Routes and Reaction Conditions
The synthesis of 3,5-DIETHYL 4-(2-ETHOXYPHENYL)-1-(4-METHYLPHENYL)-1,4-DIHYDROPYRIDINE-3,5-DICARBOXYLATE typically involves multi-step organic reactions. One common method is the Hantzsch dihydropyridine synthesis, which involves the condensation of an aldehyde, a β-keto ester, and ammonia or an amine under acidic or basic conditions. The reaction is usually carried out in a solvent such as ethanol or methanol at elevated temperatures.
Industrial Production Methods
In an industrial setting, the production of this compound may involve optimized reaction conditions to maximize yield and purity. This includes the use of high-purity reagents, controlled reaction temperatures, and efficient purification techniques such as recrystallization or chromatography.
Chemical Reactions Analysis
Types of Reactions
3,5-DIETHYL 4-(2-ETHOXYPHENYL)-1-(4-METHYLPHENYL)-1,4-DIHYDROPYRIDINE-3,5-DICARBOXYLATE can undergo various chemical reactions, including:
Oxidation: The dihydropyridine ring can be oxidized to form pyridine derivatives.
Reduction: The compound can be reduced to form tetrahydropyridine derivatives.
Substitution: The ethyl, ethoxyphenyl, and methylphenyl groups can be substituted with other functional groups under appropriate conditions.
Common Reagents and Conditions
Oxidation: Common oxidizing agents include potassium permanganate (KMnO4) and chromium trioxide (CrO3).
Reduction: Reducing agents such as lithium aluminum hydride (LiAlH4) or sodium borohydride (NaBH4) are often used.
Substitution: Substitution reactions may involve reagents like halogens (e.g., chlorine, bromine) or nucleophiles (e.g., amines, thiols).
Major Products
The major products formed from these reactions depend on the specific conditions and reagents used. For example, oxidation may yield pyridine derivatives, while reduction may produce tetrahydropyridine derivatives.
Scientific Research Applications
3,5-DIETHYL 4-(2-ETHOXYPHENYL)-1-(4-METHYLPHENYL)-1,4-DIHYDROPYRIDINE-3,5-DICARBOXYLATE has several applications in scientific research:
Medicinal Chemistry: It is studied for its potential as a calcium channel blocker, which could be useful in treating cardiovascular diseases.
Material Science: The compound’s unique structure makes it a candidate for use in organic electronic materials, such as organic light-emitting diodes (OLEDs) and organic photovoltaics (OPVs).
Biological Research: It is investigated for its interactions with biological macromolecules, which could lead to the development of new therapeutic agents.
Mechanism of Action
The mechanism of action of 3,5-DIETHYL 4-(2-ETHOXYPHENYL)-1-(4-METHYLPHENYL)-1,4-DIHYDROPYRIDINE-3,5-DICARBOXYLATE involves its interaction with calcium channels in cell membranes. By binding to these channels, the compound can inhibit the influx of calcium ions, leading to a decrease in intracellular calcium levels. This action can result in the relaxation of smooth muscle cells, particularly in the cardiovascular system, thereby reducing blood pressure.
Comparison with Similar Compounds
Similar Compounds
Nifedipine: Another dihydropyridine calcium channel blocker used in the treatment of hypertension and angina.
Amlodipine: A long-acting dihydropyridine calcium channel blocker used to manage hypertension and coronary artery disease.
Felodipine: A dihydropyridine calcium channel blocker with similar applications in cardiovascular therapy.
Uniqueness
3,5-DIETHYL 4-(2-ETHOXYPHENYL)-1-(4-METHYLPHENYL)-1,4-DIHYDROPYRIDINE-3,5-DICARBOXYLATE is unique due to its specific substituents, which may confer distinct pharmacological properties compared to other dihydropyridine derivatives. Its ethoxyphenyl and methylphenyl groups could influence its binding affinity and selectivity for calcium channels, potentially leading to different therapeutic effects and side effect profiles.
Properties
Molecular Formula |
C26H29NO5 |
---|---|
Molecular Weight |
435.5 g/mol |
IUPAC Name |
diethyl 4-(2-ethoxyphenyl)-1-(4-methylphenyl)-4H-pyridine-3,5-dicarboxylate |
InChI |
InChI=1S/C26H29NO5/c1-5-30-23-11-9-8-10-20(23)24-21(25(28)31-6-2)16-27(17-22(24)26(29)32-7-3)19-14-12-18(4)13-15-19/h8-17,24H,5-7H2,1-4H3 |
InChI Key |
QIMNPOBYSMUNDJ-UHFFFAOYSA-N |
Canonical SMILES |
CCOC1=CC=CC=C1C2C(=CN(C=C2C(=O)OCC)C3=CC=C(C=C3)C)C(=O)OCC |
Origin of Product |
United States |
Disclaimer and Information on In-Vitro Research Products
Please be aware that all articles and product information presented on BenchChem are intended solely for informational purposes. The products available for purchase on BenchChem are specifically designed for in-vitro studies, which are conducted outside of living organisms. In-vitro studies, derived from the Latin term "in glass," involve experiments performed in controlled laboratory settings using cells or tissues. It is important to note that these products are not categorized as medicines or drugs, and they have not received approval from the FDA for the prevention, treatment, or cure of any medical condition, ailment, or disease. We must emphasize that any form of bodily introduction of these products into humans or animals is strictly prohibited by law. It is essential to adhere to these guidelines to ensure compliance with legal and ethical standards in research and experimentation.