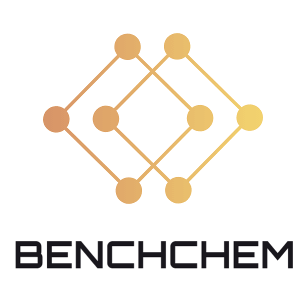
Soya oil fatty acids
- Click on QUICK INQUIRY to receive a quote from our team of experts.
- With the quality product at a COMPETITIVE price, you can focus more on your research.
Overview
Description
Synthesis Analysis
Soya oil fatty acids can be synthesized through various enzymatic processes. For example, biolubricants have been produced from soybean oil processing by-products using Candida rugosa lipase, showcasing the potential of using soybean fatty acid distillate as a source of free fatty acids for biolubricant production (Fernandes et al., 2021). Additionally, enzymatic production of trans-free plastic fats from highly hydrogenated soybean oil enriches the product with α-linolenic acid and medium-chain fatty acids, demonstrating the versatility of enzymatic processes in modifying fatty acid compositions (Zhao et al., 2013).
Molecular Structure Analysis
The molecular structure of this compound includes a variety of unsaturated and saturated fatty acids. The enzymatic synthesis methods mentioned allow for the production of structured lipids with desired fatty acid profiles, including modifications at the sn-2 position or incorporation of specific fatty acids like stearidonic acid (SDA) for enhanced nutritional or industrial properties (Teichert & Akoh, 2011).
Chemical Reactions and Properties
This compound undergo various chemical reactions, including esterification and interesterification, to produce products with specific characteristics. For instance, the enzymatic interesterification of SDA soybean oil with tripalmitin can create structured lipids with significant amounts of palmitic acid at the sn-2 position, mimicking human milk fat and providing a potential ingredient for infant formula (Teichert & Akoh, 2011).
Physical Properties Analysis
The physical properties of this compound, such as melting point, viscosity, and solid fat content, can be tailored through enzymatic processes. The production of biolubricants and plastic fats demonstrates the ability to adjust these properties to meet specific application requirements, whether for industrial use or as food ingredients (Fernandes et al., 2021); (Zhao et al., 2013).
Chemical Properties Analysis
The chemical properties of this compound, such as reactivity in polymerization and suitability for various chemical modifications, enable their use in diverse applications. For instance, the synthesis of vinyl monomers from soybean oil for free radical polymerization offers potential for sustainable materials production (Tarnavchyk et al., 2015).
Scientific Research Applications
1. Biodiesel Production
Soya oil fatty acids are utilized in biodiesel production. Umeuzuegbu J.C (2021) investigated the transesterification of used soya oil into biodiesel, focusing on the kinetics of the reaction and the cold flow properties of the resulting biodiesel. This research highlights the potential of this compound in sustainable fuel production (Umeuzuegbu, 2021).
2. Cardiovascular Health
This compound have been studied for their effects on cardiovascular health. A study by Hunter et al. (2010) compared dietary stearic acid from soybean oil with other fatty acids, finding that it beneficially affects LDL cholesterol, a primary target for cardiovascular disease risk reduction (Hunter, Zhang, & Kris-Etherton, 2010).
3. Impact on Agricultural Practices
Research by Lee et al. (2008) explored how environmental factors, like irrigation, affect the unsaturated fatty acid content in soya bean seed oil. This study provides insights into agricultural practices that can influence the fatty acid profile of soya oil (Lee, Oliva, Sleper, & Shannon, 2008).
4. Automotive Engine Performance
This compound are also being explored for their use in automotive engines. Tripathi and Subramanian (2017) conducted an experimental investigation to analyze the effect of soya soap stock based acid oil biodiesel on engine performance, noting improvements in emissions and efficiency (Tripathi & Subramanian, 2017).
Mechanism of Action
Target of Action
Soya oil fatty acids primarily target the peroxisome proliferator-activated receptor-alpha (PPAR-alpha) . PPAR-alpha is a key regulator of lipid metabolism and inflammation, and it plays a significant role in maintaining energy homeostasis .
Mode of Action
Soya oil is broken down into free fatty acids that activate PPAR-alpha . This activation regulates the hepatic CYP4A1, an enzyme that hydroxylates saturated and unsaturated fatty acids . Soya oil also prevents the up/downregulation of efflux transporters and maintains mRNA levels of hepatic antioxidant enzymes .
Biochemical Pathways
The major unsaturated fatty acids in soya oil triglycerides are the polyunsaturates alpha-linolenic acid (C-18:3), 7-10%, and linoleic acid (C-18:2), 51%; and the monounsaturate oleic acid (C-18:1), 23% . The enzymes involved in the desaturation of oleic acid to linoleic acid is ∆12 oleate desaturase (FAD2), and then linoleic acid to linolenic acid is ω-3-Fatty Acid Desaturase (FAD3) . These fatty acids are involved in various metabolic pathways, including lipid biosynthesis .
Pharmacokinetics
The pharmacokinetics of this compound involve their breakdown into free fatty acids that activate PPAR-alpha . .
Result of Action
The activation of PPAR-alpha by this compound leads to the regulation of hepatic CYP4A1 . This results in the hydroxylation of saturated and unsaturated fatty acids, which can influence various physiological processes, including inflammation and energy homeostasis . Additionally, this compound help maintain the mRNA levels of hepatic antioxidant enzymes, which can have protective effects against oxidative stress .
Action Environment
Environmental factors can significantly influence the action, efficacy, and stability of this compound. For instance, high temperatures during the seed-filling stage can affect the concentration of polyunsaturated fatty acids in soya oil . This can lead to seeds with lower concentrations of the polyunsaturated fatty acids, linoleic, and linolenic acids, and higher concentrations of oleic acid . Understanding these environmental influences can help in predicting the phenotype of this compound in given environments and estimating genotype by environment interactions .
Safety and Hazards
Future Directions
The future of soybean oil is promising. With the development of genetic modification technologies, companies are exploring ways to modify the fatty acid composition of soybean oil to improve its nutritional profile and stability . Furthermore, the market for soya fatty acids is expected to grow in the future .
properties
{ "Design of the Synthesis Pathway": "The synthesis of Soya oil fatty acids can be achieved through hydrolysis of Soya oil followed by acidification and separation of the fatty acids.", "Starting Materials": [ "Soya oil" ], "Reaction": [ "Hydrolysis of Soya oil using water and a catalyst such as sodium hydroxide or sulfuric acid", "Acidification of the hydrolyzed mixture to convert the fatty acids into their free acid form", "Separation of the fatty acids from the mixture using techniques such as distillation or extraction" ] } | |
CAS RN |
8001-22-7 |
Molecular Formula |
UNKNOWN |
Origin of Product |
United States |
Q & A
Q1: What are the key findings regarding the impact of dietary soya oil fatty acids on milk production in cows?
A1: Research indicates that feeding dairy cows with free and protected soya oil, as well as this compound, influences the fatty acid profile of their milk []. Specifically, the presence of trans-18:1 fatty acids and polyunsaturated fatty acids in the milk provides insights into rumen conditions. Additionally, changes in citrate levels in milk can be used to evaluate the impact of this compound on fatty acid synthesis within the mammary gland [].
Q2: How are this compound utilized in the synthesis of alkyd resins?
A2: this compound serve as a key component in the production of alkyd resins, particularly those used in baked enamels and polyurethane paints [, ]. These resins are synthesized through a catalytic esterification process, often employing inexpensive materials like castor oil fatty acid and crude terephthalic acid, with hypophosphorous acid acting as a catalyst []. This method offers cost advantages over traditional alkyd production using soya oil fatty acid and phthalic anhydride [].
Q3: What is the role of monoethanolamine in reactions involving this compound?
A3: Monoethanolamine reacts with this compound in the presence of an alkali catalyst to produce soya oil fatty acid monoethanolamine []. This reaction is influenced by factors such as temperature, reaction time, and catalyst concentration. For instance, using sodium methoxide (NaOCH3) as the catalyst at 130°C for 3 hours resulted in high conversion rates of monoethanolamine [].
Q4: Are there any efforts to model the transesterification process of used soya oil for biodiesel production?
A6: Researchers have developed empirical models to understand the kinetics of transesterification when producing biodiesel from used soya oil []. By applying rate laws to the three-step reversible reaction scheme, they derived forward and reverse rate constants. These models help identify the rate-limiting step and calculate activation energy, ultimately aiding in the optimization of biodiesel production processes [].
Disclaimer and Information on In-Vitro Research Products
Please be aware that all articles and product information presented on BenchChem are intended solely for informational purposes. The products available for purchase on BenchChem are specifically designed for in-vitro studies, which are conducted outside of living organisms. In-vitro studies, derived from the Latin term "in glass," involve experiments performed in controlled laboratory settings using cells or tissues. It is important to note that these products are not categorized as medicines or drugs, and they have not received approval from the FDA for the prevention, treatment, or cure of any medical condition, ailment, or disease. We must emphasize that any form of bodily introduction of these products into humans or animals is strictly prohibited by law. It is essential to adhere to these guidelines to ensure compliance with legal and ethical standards in research and experimentation.