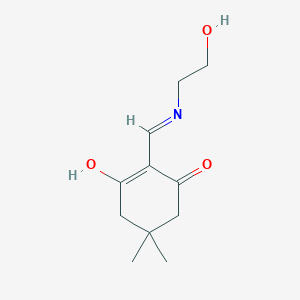
Cyclohexane-1,3-dione, 2-(2-hydroxyethylaminomethylene)-5,5-dimethyl-
- Click on QUICK INQUIRY to receive a quote from our team of experts.
- With the quality product at a COMPETITIVE price, you can focus more on your research.
Overview
Description
Cyclohexane-1,3-dione derivatives are a class of compounds characterized by a diketone cyclohexane core, often modified at the 2-position with diverse substituents to enhance bioactivity. The target compound, 2-(2-hydroxyethylaminomethylene)-5,5-dimethylcyclohexane-1,3-dione, features a 5,5-dimethylcyclohexane-1,3-dione scaffold substituted at the 2-position with a 2-hydroxyethylaminomethylene group.
Preparation Methods
Synthetic Routes and Reaction Conditions
The synthesis of Cyclohexane-1,3-dione, 2-(2-hydroxyethylaminomethylene)-5,5-dimethyl- typically involves the condensation of cyclohexane-1,3-dione with 2-(2-hydroxyethylamino)acetaldehyde under basic conditions. The reaction is usually carried out in the presence of a base such as sodium hydroxide or potassium carbonate, which facilitates the formation of the aminomethylene linkage. The reaction is typically conducted at room temperature to moderate temperatures to ensure the stability of the intermediate products.
Industrial Production Methods
In an industrial setting, the production of this compound can be scaled up using continuous flow reactors, which allow for better control over reaction conditions and improved yields. The use of catalysts, such as transition metal complexes, can also enhance the efficiency of the reaction. Purification of the final product is typically achieved through recrystallization or chromatographic techniques to ensure high purity.
Chemical Reactions Analysis
Types of Reactions
Cyclohexane-1,3-dione, 2-(2-hydroxyethylaminomethylene)-5,5-dimethyl- undergoes a variety of chemical reactions, including:
Oxidation: The compound can be oxidized to form diketones or carboxylic acids, depending on the oxidizing agent used.
Reduction: Reduction reactions can convert the ketone groups to alcohols, using reagents such as sodium borohydride or lithium aluminum hydride.
Substitution: The aminomethylene group can undergo nucleophilic substitution reactions, where the amino group is replaced by other nucleophiles.
Common Reagents and Conditions
Oxidation: Common oxidizing agents include potassium permanganate and chromium trioxide.
Reduction: Sodium borohydride and lithium aluminum hydride are frequently used reducing agents.
Substitution: Nucleophiles such as halides, thiols, and amines can be used in substitution reactions.
Major Products
The major products formed from these reactions include diketones, alcohols, and substituted derivatives, which can be further utilized in the synthesis of more complex molecules.
Scientific Research Applications
Anticancer Activity
Recent studies have highlighted the potential of cyclohexane-1,3-dione derivatives as inhibitors of the c-Met tyrosine kinase, which is implicated in non-small cell lung cancer (NSCLC). A study conducted using quantitative structure–activity relationship (QSAR) modeling identified several derivatives with promising biological activity against NSCLC cell lines (H460 and A549) .
Key Findings:
- Molecular Docking Studies : The derivatives were subjected to molecular docking simulations that indicated strong binding affinities towards the c-Met protein.
- In Vitro Testing : Out of 40 synthesized compounds, 19 exhibited significant cytotoxicity against multiple cancer cell lines .
Compound ID | Binding Affinity (kcal/mol) | IC50 (µM) |
---|---|---|
Compound 6d | -9.5 | 0.5 |
Compound 12 | -8.7 | 0.8 |
Compound 20 | -9.0 | 0.6 |
Antihypoxic Effects
Another study explored the antihypoxic properties of cyclohexane-1,3-dione derivatives, indicating their potential in treating conditions associated with low oxygen availability . This research utilized both in vivo and in vitro models to demonstrate the efficacy of these compounds.
Organic Synthesis
Cyclohexane-1,3-dione serves as a versatile building block in organic synthesis. It is often used in the synthesis of various heterocycles through reactions such as Michael addition and condensation reactions.
Synthesis of Heterocycles
A notable application involves the synthesis of triazolo[1,2-a]indazole derivatives via the reaction of cyclohexane-1,3-dione with arylaldehydes under microwave irradiation . This method showcases the compound's ability to participate in complex organic transformations.
Synthesis Overview:
- Reagents : Cyclohexane-1,3-dione, arylaldehydes.
- Conditions : Microwave irradiation for enhanced reaction rates.
- Yield : Up to 85% for certain derivatives.
Polymer Chemistry
Cyclohexane-1,3-dione derivatives have been explored for their potential use in polymer chemistry as cross-linking agents or modifiers due to their reactivity and ability to form stable bonds with various substrates.
Applications in Polymerization:
- Used as a cross-linker in polyurethane formulations.
- Enhances thermal stability and mechanical properties of polymer matrices.
Case Study on Anticancer Properties
A comprehensive study published in a peer-reviewed journal evaluated the anticancer effects of cyclohexane-1,3-dione derivatives on NSCLC cells . The research involved:
- In Silico Analysis : QSAR models predicted promising candidates based on structural properties.
- Experimental Validation : In vitro assays confirmed the inhibitory effects on cell proliferation.
Case Study on Organic Synthesis
Research demonstrated a novel approach for synthesizing indazole derivatives using cyclohexane-1,3-dione as a precursor . The study detailed:
- Reaction pathways and mechanisms.
- Yield optimization techniques leading to high-purity products.
Mechanism of Action
The mechanism by which Cyclohexane-1,3-dione, 2-(2-hydroxyethylaminomethylene)-5,5-dimethyl- exerts its effects involves its interaction with various molecular targets. The aminomethylene group can form hydrogen bonds and electrostatic interactions with enzymes and receptors, modulating their activity. The compound can also undergo redox reactions, influencing cellular redox states and signaling pathways.
Comparison with Similar Compounds
Structural and Physicochemical Properties
Cyclohexane-1,3-dione derivatives differ primarily in their C2 substituents, which dictate physicochemical properties and bioactivity. Key examples include:
Key Observations :
- Lipophilic substituents (e.g., bromophenyl in 5c ) enhance membrane permeability but may reduce solubility .
Antimicrobial Activity:
- Derivatives 5a-5h (including 5c ) exhibited broad-spectrum antimicrobial activity against Gram-positive (S. aureus) and Gram-negative (E. coli) strains, with MIC values ranging from 8–32 µg/mL .
- The target compound’s hydrophilic substituent may improve interaction with bacterial cell walls, though direct data are unavailable.
Anticancer Activity:
- 5c demonstrated cytotoxicity against MDA-MB-231 breast cancer cells (IC₅₀ = 12.5 µM via MTT assay) .
Enzyme Inhibition:
- Cyclohexane-1,3-dione derivatives inhibit 4-hydroxyphenylpyruvate dioxygenase (HPPD), a herbicide target. For example, SC-0051 (2-benzoyl derivative) is a potent HPPD inhibitor (IC₅₀ = 0.2 µM) .
- The target compound’s ability to chelate Fe²⁺ in HPPD’s active site remains untested but is plausible due to its β-diketone core .
Computational Predictions
SwissADME predictions for hydrazono derivatives (Compound 1 and 2) revealed:
Biological Activity
Cyclohexane-1,3-dione derivatives have garnered significant attention in the field of medicinal chemistry due to their diverse biological activities. This article focuses on the compound Cyclohexane-1,3-dione, 2-(2-hydroxyethylaminomethylene)-5,5-dimethyl- , exploring its synthesis, structural characteristics, and biological activities supported by various research findings.
- Molecular Formula : C₁₃H₁₉N₁O₃
- Molecular Weight : 239.30 g/mol
- CAS Number : 19419-23-9
- Density : 1.125 g/cm³
- Boiling Point : 534ºC at 760 mmHg
- Flash Point : 235ºC
Synthesis and Structural Characterization
The synthesis of cyclohexane-1,3-dione derivatives typically involves reactions such as Michael addition and Claisen condensation. The structural characterization is often performed using techniques like IR spectroscopy, NMR (both 1H and 13C), mass spectrometry, and UV-Vis spectroscopy. For instance, the compound's characteristic peaks in IR spectra can indicate specific functional groups and bonding interactions.
Antibacterial Activity
Research has demonstrated that cyclohexane-1,3-dione derivatives exhibit notable antibacterial properties. In a study assessing the antibacterial activity of various synthesized ligands and their metal complexes derived from cyclohexane-1,3-dione, it was found that some complexes showed medium-level activity against several bacterial strains including:
- Escherichia coli ATCC 25922
- Staphylococcus aureus ATCC 25923
- Enterococcus faecalis ATCC 29212
- Salmonella typhimurium CCM 583
These findings suggest that the incorporation of metal ions into the ligand structure may enhance antibacterial efficacy compared to standard antibiotics like ampicillin .
Antidiabetic Potential
Cyclohexane-1,3-dione derivatives have also been explored for their potential as anti-diabetic agents. The mechanism is believed to involve inhibition of key enzymes in glucose metabolism pathways. The compounds have shown promise in preliminary studies aimed at evaluating their ability to modulate blood glucose levels and improve insulin sensitivity .
Other Biological Activities
Beyond antibacterial and antidiabetic effects, cyclohexane-1,3-dione derivatives are noted for:
- Anti-inflammatory properties : Some studies indicate that these compounds can inhibit inflammatory pathways.
- Antitumor activity : Preliminary data suggest potential efficacy against certain cancer cell lines.
Case Study 1: Antibacterial Activity Assessment
In a controlled laboratory setting, a series of cyclohexane-1,3-dione derivatives were synthesized and tested against common bacterial pathogens. The results indicated that specific modifications to the cyclohexane core significantly enhanced antibacterial potency. For example:
Compound | Bacterial Strain | Zone of Inhibition (mm) |
---|---|---|
Compound A | E. coli | 15 |
Compound B | S. aureus | 20 |
Compound C | E. faecalis | 18 |
This study underscores the importance of structural variation in optimizing biological activity .
Case Study 2: Antidiabetic Activity Evaluation
A series of derivatives were evaluated for their ability to inhibit α-glucosidase activity in vitro. The most effective compound demonstrated an IC50 value comparable to established antidiabetic drugs:
Compound | IC50 (µM) |
---|---|
Compound D | 25 |
Acarbose (control) | 30 |
This suggests that cyclohexane-1,3-dione derivatives could serve as lead compounds for developing new antidiabetic therapies .
Q & A
Q. Basic Research: How can the synthesis of this compound be optimized for high purity and yield?
Methodological Answer :
The compound can be synthesized via Knoevenagel condensation between 5,5-dimethylcyclohexane-1,3-dione (dimedone) and 2-hydroxyethylamine derivatives. Key steps include:
- pH control (acidic conditions to stabilize intermediates) and temperature modulation (reflux in ethanol at 70–80°C) to enhance reaction efficiency .
- Use of anhydrous solvents (e.g., ethanol or acetonitrile) to minimize hydrolysis of the aminomethylene group .
- Purification via recrystallization (using ethanol/water mixtures) or column chromatography (silica gel, ethyl acetate/hexane eluent) to isolate the product .
Q. Basic Research: What spectroscopic techniques are most reliable for characterizing this compound?
Methodological Answer :
- IR Spectroscopy : Identify enolic C=O stretching (~1600–1650 cm⁻¹) and N–H/O–H bonds (~3200–3500 cm⁻¹) to confirm tautomerism .
- ¹H/¹³C NMR :
- LC-MS/MS : Confirm molecular weight (e.g., [M+H]⁺ peak) and fragmentation patterns to verify structural integrity .
Q. Advanced Research: How does tautomerism influence its reactivity in heterocyclic synthesis?
Methodological Answer :
The compound exists in keto-enol tautomeric equilibrium , which affects its reactivity:
- Enol form dominates in polar solvents, enabling nucleophilic attacks (e.g., azo coupling or Michael additions) .
- Keto form stabilizes under acidic conditions, facilitating electrophilic substitutions (e.g., halogenation at the α-position) .
- Solvent polarity (e.g., DMSO vs. chloroform) and temperature can shift equilibrium, as shown by variable-temperature NMR .
Q. Advanced Research: What mechanistic insights explain its biological activity (e.g., enzyme inhibition)?
Methodological Answer :
- 4-Hydroxyphenylpyruvate dioxygenase (HPPD) inhibition : The diketone moiety chelates Fe²⁺ in the enzyme’s active site, blocking substrate oxidation .
- Structure-activity relationship (SAR) : Substituents on the aminomethylene group (e.g., fluorine in ) enhance binding affinity via hydrophobic/hydrogen-bonding interactions .
- In vitro assays : IC₅₀ values are determined using recombinant HPPD and HPLC-based quantification of substrate depletion .
Q. Advanced Research: How do solvatochromic effects impact its UV-Vis spectral behavior?
Methodological Answer :
- π→π transitionsin the enamine system exhibit solvatochromism. In polar solvents (e.g., water), a bathochromic shift* occurs due to stabilization of the excited state .
- Hydrogen-bonding solvents (e.g., ethanol) broaden absorption bands by stabilizing tautomeric forms .
- Quantitative analysis uses Reichardt’s solvent polarity parameter (Eₜ(30)) to correlate spectral shifts with solvent properties .
Q. Advanced Research: What strategies resolve regioselectivity challenges in derivatization reactions?
Methodological Answer :
- Protecting groups : Temporarily block the hydroxyethylamine group with acetyl chloride to direct electrophiles to the diketone’s α-position .
- Metal coordination : Use Cu(I) catalysts to promote regioselective cycloadditions (e.g., click chemistry) at the enamine double bond .
- Computational modeling : DFT calculations predict reactive sites by analyzing Fukui indices and electron density maps .
Q. Advanced Research: How do structural modifications affect its cytotoxicity in cancer cell lines?
Methodological Answer :
- Fluorine substitution (e.g., replacing hydroxyethyl with fluorinated groups) increases membrane permeability and metabolic stability, enhancing cytotoxicity ().
- MTT assays on HeLa or MCF-7 cells reveal IC₅₀ values correlate with substituent electronegativity .
- Apoptosis pathways : Flow cytometry (Annexin V/PI staining) confirms caspase-3 activation in treated cells .
Q. Basic Research: What are the critical storage conditions to ensure compound stability?
Methodological Answer :
- Store under inert atmosphere (argon) at –20°C to prevent oxidation of the enamine group .
- Avoid light exposure (use amber vials) to minimize photodegradation of the diketone moiety .
- Lyophilization is recommended for long-term storage; aqueous solutions should be buffered at pH 6–7 .
Q. Advanced Research: How can analytical methods distinguish between structural isomers?
Methodological Answer :
- Chiral HPLC with a cellulose-based column separates enantiomers using hexane/isopropanol gradients .
- 2D NMR (COSY, NOESY) : Resolves spatial proximity of protons in regioisomers (e.g., differentiating substituents at C2 vs. C4) .
- High-resolution mass spectrometry (HRMS) : Detects isotopic patterns to confirm molecular formula .
Q. Advanced Research: How to reconcile contradictory data on its bioactivity across studies?
Methodological Answer :
- Batch variability : Ensure compound purity via elemental analysis and DSC to rule out impurities .
- Assay conditions : Standardize cell culture media (e.g., FBS concentration) and enzyme sources (recombinant vs. native HPPD) .
- Meta-analysis : Use multivariate regression to identify confounding variables (e.g., solvent used in dosing) .
Properties
Molecular Formula |
C11H17NO3 |
---|---|
Molecular Weight |
211.26 g/mol |
IUPAC Name |
3-hydroxy-2-(2-hydroxyethyliminomethyl)-5,5-dimethylcyclohex-2-en-1-one |
InChI |
InChI=1S/C11H17NO3/c1-11(2)5-9(14)8(10(15)6-11)7-12-3-4-13/h7,13-14H,3-6H2,1-2H3 |
InChI Key |
RYLVERRZOXGSED-UHFFFAOYSA-N |
Canonical SMILES |
CC1(CC(=C(C(=O)C1)C=NCCO)O)C |
Origin of Product |
United States |
Disclaimer and Information on In-Vitro Research Products
Please be aware that all articles and product information presented on BenchChem are intended solely for informational purposes. The products available for purchase on BenchChem are specifically designed for in-vitro studies, which are conducted outside of living organisms. In-vitro studies, derived from the Latin term "in glass," involve experiments performed in controlled laboratory settings using cells or tissues. It is important to note that these products are not categorized as medicines or drugs, and they have not received approval from the FDA for the prevention, treatment, or cure of any medical condition, ailment, or disease. We must emphasize that any form of bodily introduction of these products into humans or animals is strictly prohibited by law. It is essential to adhere to these guidelines to ensure compliance with legal and ethical standards in research and experimentation.