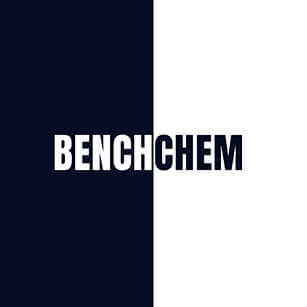
Serpentinic acid
- Click on QUICK INQUIRY to receive a quote from our team of experts.
- With the quality product at a COMPETITIVE price, you can focus more on your research.
Overview
Description
Serpentinic acid is a naturally occurring compound found in various plants, particularly within the family Apocynaceae It is a terpene indole alkaloid, known for its complex structure and significant biological activities
Biochemical Analysis
Biochemical Properties
Serpentinic acid plays a crucial role in various biochemical reactions. It interacts with several enzymes, proteins, and other biomolecules. For instance, this compound is known to inhibit the enzyme acetylcholinesterase, which is involved in the breakdown of the neurotransmitter acetylcholine . This inhibition can lead to increased levels of acetylcholine in the synaptic cleft, enhancing cholinergic transmission. Additionally, this compound interacts with various receptors, including the serotonin and dopamine receptors, influencing neurotransmission and mood regulation .
Cellular Effects
This compound has profound effects on various types of cells and cellular processes. It influences cell function by modulating cell signaling pathways, gene expression, and cellular metabolism. For example, this compound has been shown to activate the MAPK/ERK signaling pathway, which plays a critical role in cell proliferation, differentiation, and survival . Furthermore, this compound can alter gene expression by binding to specific transcription factors, leading to changes in the expression of genes involved in cell cycle regulation and apoptosis . It also affects cellular metabolism by modulating the activity of key metabolic enzymes, thereby influencing energy production and utilization .
Molecular Mechanism
The molecular mechanism of this compound involves several key interactions at the molecular level. This compound exerts its effects by binding to specific biomolecules, leading to enzyme inhibition or activation and changes in gene expression. For instance, this compound binds to the active site of acetylcholinesterase, inhibiting its activity and preventing the breakdown of acetylcholine . This binding interaction is facilitated by hydrogen bonding and hydrophobic interactions between this compound and the enzyme’s active site residues . Additionally, this compound can activate or inhibit various signaling pathways by binding to receptors or other signaling molecules, thereby modulating cellular responses .
Temporal Effects in Laboratory Settings
In laboratory settings, the effects of this compound can change over time. The stability and degradation of this compound are critical factors that influence its long-term effects on cellular function. Studies have shown that this compound is relatively stable under physiological conditions, with minimal degradation over time . Prolonged exposure to this compound can lead to adaptive changes in cellular responses, such as desensitization of receptors or upregulation of compensatory pathways . These temporal effects are important considerations for the use of this compound in experimental and therapeutic applications .
Dosage Effects in Animal Models
The effects of this compound vary with different dosages in animal models. At low doses, this compound can enhance cognitive function and improve mood by modulating neurotransmitter levels and receptor activity . At high doses, this compound can exhibit toxic or adverse effects, such as neurotoxicity and hepatotoxicity . These threshold effects highlight the importance of careful dosage optimization in preclinical and clinical studies to maximize the therapeutic benefits of this compound while minimizing potential risks .
Metabolic Pathways
This compound is involved in several metabolic pathways, interacting with various enzymes and cofactors. One of the key metabolic pathways involving this compound is its conversion to serpentine, a related alkaloid, through enzymatic reactions . This conversion is catalyzed by specific enzymes, such as methyltransferases, which add a methyl group to this compound, resulting in the formation of serpentine . Additionally, this compound can influence metabolic flux and metabolite levels by modulating the activity of key metabolic enzymes, such as those involved in glycolysis and the tricarboxylic acid cycle .
Transport and Distribution
The transport and distribution of this compound within cells and tissues are mediated by specific transporters and binding proteins. This compound can be transported across cell membranes through active transport mechanisms, involving transporters such as organic cation transporters . Once inside the cell, this compound can bind to intracellular proteins, such as cytosolic enzymes and receptors, influencing its localization and accumulation . The distribution of this compound within tissues is also influenced by factors such as blood flow, tissue permeability, and binding affinity to plasma proteins .
Subcellular Localization
The subcellular localization of this compound is critical for its activity and function. This compound can be localized to specific cellular compartments or organelles through targeting signals or post-translational modifications . For example, this compound can be directed to the endoplasmic reticulum or mitochondria, where it can interact with specific enzymes and receptors . The subcellular localization of this compound can influence its activity by modulating its access to target biomolecules and its involvement in specific cellular processes .
Preparation Methods
Synthetic Routes and Reaction Conditions: The synthesis of serpentinic acid typically involves the extraction from plant sources, followed by purification processes. One common method includes the use of organic solvents to extract the compound from plant tissues, followed by chromatographic techniques to isolate and purify the acid.
Industrial Production Methods: Industrial production of this compound often involves large-scale extraction from plants such as Catharanthus roseus and Rauvolfia serpentina. The process includes:
- Harvesting plant material.
- Drying and grinding the material.
- Using solvents like methanol or ethanol for extraction.
- Employing chromatographic methods for purification.
Chemical Reactions Analysis
Types of Reactions: Serpentinic acid undergoes various chemical reactions, including:
Oxidation: This reaction can lead to the formation of different oxidized derivatives.
Reduction: Reduction reactions can modify the functional groups within the molecule.
Substitution: Substitution reactions can occur at various positions on the indole ring.
Common Reagents and Conditions:
Oxidation: Common oxidizing agents include potassium permanganate and hydrogen peroxide.
Reduction: Reducing agents such as sodium borohydride and lithium aluminum hydride are often used.
Substitution: Halogenating agents and nucleophiles are typically employed in substitution reactions.
Major Products: The major products formed from these reactions depend on the specific conditions and reagents used. For example, oxidation can yield hydroxylated or carboxylated derivatives, while reduction can produce amine or alcohol derivatives.
Scientific Research Applications
Serpentinic acid has a wide range of applications in scientific research:
Chemistry: It is used as a precursor for synthesizing various complex organic molecules.
Biology: Studies have shown its potential in modulating biological pathways and interactions.
Medicine: It exhibits pharmacological properties, including anti-inflammatory and anticancer activities.
Industry: It is used in the development of new drugs and therapeutic agents.
Mechanism of Action
The mechanism of action of serpentinic acid involves its interaction with specific molecular targets and pathways. It is known to:
- Bind to certain receptors in the body, modulating their activity.
- Inhibit or activate enzymes involved in metabolic pathways.
- Interfere with the replication and transcription processes in cells, leading to its anticancer effects.
Comparison with Similar Compounds
Serpentinic acid is unique compared to other similar compounds due to its specific structure and biological activities. Some similar compounds include:
- Tetrahydroalstonine
- Rauvotetraphylline E
- Alstonine
- Akuammigine
- Mayumbine
- Serpentinine
- Ajmalicine
These compounds share structural similarities but differ in their specific functional groups and biological activities. This compound stands out due to its potent pharmacological properties and diverse applications in scientific research.
Properties
CAS No. |
605-14-1 |
---|---|
Molecular Formula |
C17H17NO4 |
Appearance |
C20H19N2O3 |
Origin of Product |
United States |
Q1: What are the potential applications of silica derived from serpentine?
A: Silica from serpentine acid leaching residue shows promise as a filler material. Research indicates it can be incorporated into asphalt to potentially enhance its high-temperature performance, low-temperature properties, and aging resistance []. The amorphous structure, nearly spherical shape, and average pore size of 1.28 μm [] make it a suitable candidate for various applications, potentially including rubber reinforcement, plastics, and concrete.
Q2: How does the sulfuric acid leaching process of serpentine work, and how can it be optimized?
A: Sulfuric acid leaching extracts magnesium from serpentine, leaving behind silica. Research demonstrates that employing microwave radiation significantly enhances the leaching rate compared to traditional methods []. Optimization involves controlling factors like microwave power, radiation time, acid concentration, solid-to-liquid ratio, and particle size []. This method allows for a high yield of magnesium oxide (up to 97%) and produces amorphous silica with a composition similar to precipitated white carbon black [].
Q3: Can serpentine be utilized to produce high-purity magnesium compounds? What are the processes involved?
A: Yes, serpentine serves as a viable source for synthesizing high-purity magnesium hydroxide (Mg(OH)2) and magnesium carbonate compounds like 4MgCO3·Mg(OH)2·4H2O []. The process involves several steps:
- Purification: Impurities like iron (Fe), aluminum (Al), chromium (Cr), nickel (Ni), cobalt (Co), and manganese (Mn) are removed from the leaching solution through a combination of oxidation precipitation and sulfide precipitation methods [].
- Precipitation: The purified magnesium sulfate (MgSO4) solution is treated with ammonia (NH3·H2O) to precipitate Mg(OH)2. Subsequent precipitation with ammonium bicarbonate (NH4HCO3) yields 4MgCO3·Mg(OH)2·4H2O [].
- Refinement: The Mg(OH)2 can be further treated with diluted sodium hydroxide (NaOH) to remove residual sulfur, resulting in a high purity product (98.48% purity with 0.28% sulfur content) [].
Disclaimer and Information on In-Vitro Research Products
Please be aware that all articles and product information presented on BenchChem are intended solely for informational purposes. The products available for purchase on BenchChem are specifically designed for in-vitro studies, which are conducted outside of living organisms. In-vitro studies, derived from the Latin term "in glass," involve experiments performed in controlled laboratory settings using cells or tissues. It is important to note that these products are not categorized as medicines or drugs, and they have not received approval from the FDA for the prevention, treatment, or cure of any medical condition, ailment, or disease. We must emphasize that any form of bodily introduction of these products into humans or animals is strictly prohibited by law. It is essential to adhere to these guidelines to ensure compliance with legal and ethical standards in research and experimentation.