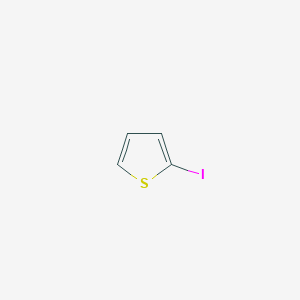
2-Iodothiophene
Overview
Description
2-Iodothiophene (C₄H₃IS, CAS 3437-95-4) is a heteroaromatic compound featuring a thiophene ring substituted with an iodine atom at the 2-position. It is a yellow to yellow-brown liquid with a density of 2.06–2.075 g/mL at 20°C and high solubility in methanol and ether . This compound serves as a versatile building block in organic synthesis, particularly in palladium-catalyzed cross-coupling reactions (e.g., Suzuki, Sonogashira, and Heck couplings) to construct complex heterocycles and functionalized thiophene derivatives . Its reactivity stems from the polarization of the C–I bond, which facilitates oxidative addition to transition metal catalysts. Applications span pharmaceuticals, materials science (e.g., conductive polymers), and agrochemicals .
Preparation Methods
Halogen Exchange via Nucleophilic Aromatic Substitution
The most well-documented synthesis of 2-iodothiophene involves nucleophilic displacement of 2-chlorothiophene using sodium iodide (NaI). This method, developed by Shijiazhuang Wanye Chemical Industry Co., Ltd., proceeds under optimized conditions :
Reaction Conditions and Mechanism
-
Substrate : 2-Chlorothiophene
-
Nucleophile : Sodium iodide (1.1 equivalents)
-
Solvent System : Acetone/tetrahydrofuran (THF) (3:1 v/v)
-
Temperature : 25°C
-
Atmosphere : Nitrogen (degassed)
-
Reaction Time : 3 hours
The reaction follows a nucleophilic aromatic substitution (SNAr) mechanism, facilitated by the electron-withdrawing sulfur atom in thiophene, which activates the 2-position for displacement. Polar aprotic solvents (acetone/THF) enhance iodide nucleophilicity, while inert conditions prevent oxidation.
Workup and Yield
Post-reaction, the organic layer is separated, and the aqueous phase is extracted with toluene. Solvent removal under reduced pressure (73°C, 0.095 MPa) yields this compound with:
Key Advantage : High regioselectivity and scalability.
Photochemical Stability and Synthesis Considerations
Photolysis studies reveal critical stability data for this compound :
Decomposition Kinetics
-
Solvents Tested : n-Heptane, dichloromethane, methanol
-
Light Exposure : UV irradiation induces C–I bond cleavage, yielding thiophene and iodine.
-
Rate Law : First-order kinetics with rate constants:
Solvent Rate Constant (k, h⁻¹) n-Heptane 0.12 Dichloromethane 0.11 Methanol 0.10
Implications for Synthesis :
-
Light Protection : Mandatory during reactions and storage.
-
Solvent Choice : Non-polar solvents (n-heptane) slightly accelerate decomposition, favoring inert atmospheres.
Alternative Iodination Strategies
Emerging methods from aromatic iodination literature suggest adaptable approaches :
Radical-Initiated Iodination
-
Promoter : trans-3,5-Dihydroperoxy-3,5-dimethyl-1,2-dioxolane
-
Regioselectivity : Enhanced para/ortho ratios in phenolic systems, potentially applicable to thiophene with modification.
Microwave-Assisted Reactions
-
Efficiency : Reduced reaction times (e.g., 30 minutes for Sonogashira couplings ).
-
Potential Application : Accelerating SNAr or direct iodination steps.
Comparative Analysis of Methods
Method | Yield (%) | Purity (%) | Conditions | Scalability |
---|---|---|---|---|
Halogen Exchange | 92.31 | 98.69 | Mild (25°C, N₂) | High |
Direct Iodination | 90* | N/A | Aqueous, 50°C | Moderate |
Photochemical | N/A | N/A | UV light, ambient temperature | Low |
*Reported for 2,5-diiodothiophene.
Chemical Reactions Analysis
Photodissociation Reactions
UV irradiation of 2-iodothiophene induces C–I bond cleavage, generating reactive intermediates:
Primary Pathway :
Secondary Products : Thiophene () and iodine () via radical recombination .
Key Findings:
-
Solvent Dependence :
-
Mechanistic Insights :
Solvent | Rate Constant (, h⁻¹) | Quantum Yield () | Major Products |
---|---|---|---|
n-Heptane | 0.018 | 0.12 (consumption) | Thiophene, |
Dichloromethane | 0.023 | N/A | Thiophene, |
Methanol | 0.035 | N/A | Thiophene, |
Cross-Coupling Reactions
This compound participates in transition-metal-catalyzed couplings for synthesizing complex heterocycles:
Sonogashira Coupling
Reaction :
Conditions :
-
Catalyst: Palladium(II) acetate/copper(I) iodide
-
Solvent: DMF
-
Microwave irradiation (rapid homogeneous phase)
Copper-Catalyzed N-Arylation
Reaction with Anilines :
Conditions :
-
Catalyst: Activated copper powder
-
Solvent: Dibutyl ether
Palladium-Catalyzed Silanolate Coupling
Reaction :
Mechanism :
-
Zero-order dependence on silanolate concentration.
-
Rate-limiting step: Oxidative addition of aryl iodide to Pd(0) .
Reaction Type | Catalyst | Conditions | Yield |
---|---|---|---|
Sonogashira | Pd/Cu | Microwave, DMF | 85–90% |
N-Arylation | Cu | Reflux, Bu₂O | <30% |
Silanolate Coupling | Pd(0) | 70°C, DMF | 75% |
Thermal Decomposition
At elevated temperatures, this compound undergoes C–I bond homolysis:
Pathway :
Products :
Scientific Research Applications
2-Iodothiophene has a wide range of applications in scientific research:
Chemistry: It is used as a building block in the synthesis of more complex organic molecules and polymers.
Medicine: Research is ongoing into its potential use in the development of new drugs and therapeutic agents.
Industry: It is used in the production of conductive polymers and materials for electronic devices.
Mechanism of Action
The mechanism by which 2-iodothiophene exerts its effects is primarily through its participation in various chemical reactions. In cross-coupling reactions, for example, the iodine atom is replaced by a different substituent, forming new carbon-carbon bonds. The molecular targets and pathways involved depend on the specific reaction and the reagents used .
Comparison with Similar Compounds
Reactivity in Cross-Coupling Reactions
2-Iodothiophene vs. Aryl Iodides (e.g., Iodobenzene, 4-Iodotoluene)
- Reaction Rate : this compound reacts faster than brominated analogs (e.g., 2-bromothiophene) due to the weaker C–I bond (bond dissociation energy ~50 kcal/mol for aryl iodides vs. ~70 kcal/mol for aryl bromides) . However, iodobenzene derivatives (e.g., 4-iodoanisole) exhibit comparable or faster reaction rates in palladium-catalyzed couplings, depending on electronic effects. For example, electron-rich 4-iodoanisole achieves 88% yield in Pd-catalyzed tandem reactions, whereas this compound yields 80% under similar conditions .
- Steric Effects : Ortho-substituted aryl iodides (e.g., o-iodoanisole) show reduced reactivity due to steric hindrance, whereas this compound’s planar thiophene ring minimizes steric constraints, enabling efficient coupling .
This compound vs. Heteroaryl Iodides (e.g., 4-Iodopyridine)
- Substrate Compatibility: this compound and 4-iodopyridine are both effective in Sonogashira and Mizoroki–Heck reactions. SCE) and facile C–I bond cleavage .
- Yield Trends : In Pd-catalyzed carbonylative difunctionalization, this compound delivers moderate to high yields (75–85%), comparable to iodobenzofuran but lower than iodocarbazoles (e.g., 3-iodo-9-phenyl-carbazole: 89%) .
Electronic and Steric Properties
Electronic Effects
- Electron-Withdrawing vs. Donating Groups : Unlike electron-deficient heteroaryl iodides (e.g., 2-iodofluorobenzene), this compound’s sulfur atom provides mild electron-withdrawing character, balancing reactivity and stability. This property makes it suitable for reactions requiring moderate electrophilicity, such as tandem carboetherification-hydrogenation (87% yield, 87% ee) .
- Comparison with Halogenated Thiophenes : 2-Bromothiophene and 2-chlorothiophene are less reactive in cross-couplings due to stronger C–Br and C–Cl bonds. For instance, Pd-catalyzed homocoupling of this compound proceeds quantitatively in 4 hours, whereas brominated analogs require prolonged reaction times .
Advantages of this compound
- Versatility: Used in synthesizing benzo[b]thiophenes (via Pd-catalyzed coupling with alkynes, 70–85% yield) and BODIPY dyes (moderate yields via Sonogashira coupling) .
- Radical Reactions : Superior performance in aryl radical-mediated NHC catalysis due to low bond dissociation energy (C–I BDE: ~45 kcal/mol) and high reducibility .
Limitations
- Competing Homocoupling : In Pd-catalyzed reactions with styrene, homocoupling byproducts (e.g., biphenyl derivatives) are observed, necessitating optimized conditions .
- Substrate Scope: Less effective in copper-free Sonogashira couplings with aliphatic alkynes, where iodobenzene derivatives dominate .
Data Tables
Table 1: Reactivity Comparison in Palladium-Catalyzed Reactions
Table 2: Physical and Electronic Properties
Biological Activity
2-Iodothiophene is a halogenated thiophene derivative that has garnered attention for its potential biological activities, particularly in the fields of medicinal chemistry and photochemistry. This article explores the biological activity of this compound, focusing on its antiproliferative effects, photochemical properties, and the mechanisms underlying its biological interactions.
Chemical Structure and Properties
This compound is characterized by a thiophene ring substituted with an iodine atom at the second position. Its molecular formula is , and it exhibits unique reactivity due to the presence of the iodine atom, which can facilitate various chemical transformations.
Antiproliferative Activity
Recent studies have highlighted the antiproliferative effects of this compound and related compounds against various cancer cell lines. For instance, research indicated that several iodothiophenes demonstrated significant growth inhibition in A2058 melanoma cells, with some compounds achieving over 60% inhibition at specific concentrations. The structure-activity relationship (SAR) studies suggest that the presence of iodine enhances the biological activity of these compounds.
Table 1: Antiproliferative Activity of Iodothiophenes
Compound | Cell Line | % Growth Inhibition | Concentration (µM) |
---|---|---|---|
This compound | A2058 | 60-70% | 10 |
5-Methyl-2-iodothiophene | A2058 | 50% | 15 |
Benzothieno[2,3-b]quinoline | A2058 | 65% | 5 |
The photochemical behavior of this compound has been extensively studied. It undergoes photoinduced reactions that lead to the formation of thiophene and iodine as primary products. The quantum yields for these reactions vary depending on the solvent used, indicating the influence of solvent polarity on the photochemical pathways.
Table 2: Photochemical Reactivity of this compound
Solvent | Quantum Yield (Φ) | Main Products |
---|---|---|
n-Heptane | 0.45 | Thiophene, Iodine |
Dichloromethane | 0.38 | Thiophene, Iodine |
Methanol | 0.50 | Thiophene, Iodine |
The biological activity of this compound can be attributed to several mechanisms:
- Cytotoxicity : The compound exhibits cytotoxic effects on cancer cells, which may be mediated through apoptosis or necrosis pathways.
- Photodynamic Activity : Upon UV irradiation, this compound generates reactive species that can induce oxidative stress in cells, leading to cell death.
- Radical Formation : The photolysis of this compound produces thienyl radicals that can participate in further chemical reactions or interact with cellular components.
Case Study 1: Antiproliferative Effects in Melanoma Cells
A study evaluated the effects of various iodothiophenes on A2058 melanoma cells. The results indicated that compounds with higher electron-withdrawing substituents exhibited increased antiproliferative activity compared to those with electron-donating groups.
Case Study 2: Photochemical Behavior in Different Solvents
Research investigating the photochemical properties of this compound revealed that solvent choice significantly affects reaction outcomes. In n-heptane, a higher quantum yield was observed compared to methanol, suggesting solvent interactions play a crucial role in photoreactivity.
Q & A
Basic Question: What are the established synthetic routes for 2-iodothiophene, and how can reaction conditions be optimized for yield and purity?
Methodological Answer:
this compound is typically synthesized via direct iodination of thiophene using iodine (I₂) in the presence of mercury(II) oxide (HgO) as a catalyst. The reaction is carried out in dioxane at elevated temperatures (60–80°C) . To optimize yield, stoichiometric control of HgO (1.5–2.0 equivalents) and rigorous exclusion of moisture are critical. Post-synthesis purification involves successive recrystallization from aqueous solutions and ligroin-benzene mixtures to achieve >98% purity . Kinetic monitoring using GC-MS is recommended to track intermediate formation and minimize byproducts like di-iodinated derivatives.
Basic Question: What analytical techniques are most effective for characterizing this compound’s electronic structure and purity?
Methodological Answer:
High-resolution photoelectron spectroscopy (PES) with HeI radiation (21.2 eV) is critical for mapping valence-shell ionization energies. For this compound, the π₁-orbital ionization energy is experimentally observed at 9.2 eV, with satellite states arising from electron correlation effects . UV-Vis spectroscopy in the gas phase reveals near-ultraviolet absorption bands (250–300 nm) linked to C-I bond dissociation dynamics, which can be further analyzed using velocity map imaging to resolve photofragment angular distributions . For purity assessment, combine NMR (¹H/¹³C) with elemental analysis (C, H, S, I) to confirm stoichiometry.
Advanced Question: How do relativistic effects influence the valence-shell ionization dynamics of this compound compared to lighter halothiophenes?
Methodological Answer:
Relativistic effects in this compound significantly alter spin-orbit coupling and ionization energies due to the heavy iodine atom. Third-order algebraic–diagrammatic construction (ADC(3)) calculations show a 0.8 eV reduction in the π₁-orbital ionization energy compared to non-relativistic models, consistent with experimental PES data . Comparative studies with 2-chloro- and 2-bromothiophene reveal a linear trend in satellite state intensities, attributed to increasing relativistic contributions across the halogen series. Synchrotron-based angle-resolved PES further distinguishes σ- and π-orbital contributions via asymmetry parameter analysis .
Advanced Question: What mechanistic insights govern palladium-catalyzed cross-coupling reactions of this compound with organosilanols?
Methodological Answer:
In fluoride-free Pd-catalyzed cross-couplings, kinetic analysis reveals a first-order dependence on both this compound and the organosilanolate. The rate-determining step involves oxidative addition of Pd⁰ into the C-I bond, followed by transmetalation with the silanolate. Key intermediates, such as Pd(II)-thienyl complexes, have been characterized via in situ Raman spectroscopy . Experimental rate equations (e.g., rate = k[Pd][2-Iodo][Silanolate]) align with a concerted mechanism, where steric effects from the thiophene ring accelerate reductive elimination .
Advanced Question: How does the reactivity of this compound compare to 3-iodothiophene in N-heterocyclic carbene (NHC) catalysis?
Methodological Answer:
this compound exhibits ~40% higher reactivity than 3-iodothiophene in NHC-mediated arylations due to reduced steric hindrance and enhanced electron-withdrawing effects at the 2-position. Density Functional Theory (DFT) studies show a lower activation barrier (ΔΔG‡ = 6.8 kcal/mol) for oxidative addition with this compound, correlating with experimental yields (82% vs. 48% for 3-iodo) . Substrate scope evaluations further indicate tolerance for electron-deficient heteroarenes (e.g., thiazoles) when using this compound .
Advanced Question: What experimental and computational strategies resolve contradictions in reported photodissociation pathways of this compound under UV irradiation?
Methodological Answer:
Conflicting reports on C-I vs. C-S bond cleavage under UV light (265–280 nm) are resolved using core-to-valence transient absorption spectroscopy . Time-resolved data (<100 fs resolution) confirm C-I bond fission as the primary pathway (quantum yield Φ = 0.74), with minor C-S cleavage (<5%) attributed to hot-band transitions . Coupled-cluster singles and doubles (CCSD) simulations reproduce the bifurcation dynamics, highlighting the role of conical intersections between ¹πσ* and ground states .
Advanced Question: How can vibrational fine structure in the photoelectron spectrum of this compound inform its excited-state dynamics?
Methodological Answer:
Vibrational progression in the 2A'' and 2A' electronic states (observed at 9.5–10.5 eV) is analyzed via Franck-Condon simulations using harmonic oscillator approximations. The 2A'' state exhibits a progression in the C-I stretching mode (ν = 450 cm⁻¹), while the 2A' state shows coupling to ring deformation modes. Temperature-dependent PES (20–300 K) quantifies mode-specific vibronic coupling, critical for modeling non-adiabatic transitions in ultrafast photochemistry .
Advanced Question: What methodologies address challenges in stereospecific functionalization of this compound for complex heterocycle synthesis?
Methodological Answer:
Chiral aldehyde-mediated asymmetric bifunctionalization enables stereocontrol in Pd-catalyzed couplings. For example, this compound reacts with amino acids via a syn-addition pathway (dr > 20:1) when using L-proline-derived aldehydes as chiral inducers . In situ X-ray absorption spectroscopy (XAS) tracks Pd oxidation states, confirming ligand-accelerated catalysis. Computational docking studies (AutoDock Vina) optimize ligand-substrate interactions to enhance enantioselectivity (er up to 97:3) .
Properties
IUPAC Name |
2-iodothiophene | |
---|---|---|
Source | PubChem | |
URL | https://pubchem.ncbi.nlm.nih.gov | |
Description | Data deposited in or computed by PubChem | |
InChI |
InChI=1S/C4H3IS/c5-4-2-1-3-6-4/h1-3H | |
Source | PubChem | |
URL | https://pubchem.ncbi.nlm.nih.gov | |
Description | Data deposited in or computed by PubChem | |
InChI Key |
ROIMNSWDOJCBFR-UHFFFAOYSA-N | |
Source | PubChem | |
URL | https://pubchem.ncbi.nlm.nih.gov | |
Description | Data deposited in or computed by PubChem | |
Canonical SMILES |
C1=CSC(=C1)I | |
Source | PubChem | |
URL | https://pubchem.ncbi.nlm.nih.gov | |
Description | Data deposited in or computed by PubChem | |
Molecular Formula |
C4H3IS | |
Source | PubChem | |
URL | https://pubchem.ncbi.nlm.nih.gov | |
Description | Data deposited in or computed by PubChem | |
DSSTOX Substance ID |
DTXSID0063026 | |
Record name | Thiophene, 2-iodo- | |
Source | EPA DSSTox | |
URL | https://comptox.epa.gov/dashboard/DTXSID0063026 | |
Description | DSSTox provides a high quality public chemistry resource for supporting improved predictive toxicology. | |
Molecular Weight |
210.04 g/mol | |
Source | PubChem | |
URL | https://pubchem.ncbi.nlm.nih.gov | |
Description | Data deposited in or computed by PubChem | |
CAS No. |
3437-95-4 | |
Record name | 2-Iodothiophene | |
Source | CAS Common Chemistry | |
URL | https://commonchemistry.cas.org/detail?cas_rn=3437-95-4 | |
Description | CAS Common Chemistry is an open community resource for accessing chemical information. Nearly 500,000 chemical substances from CAS REGISTRY cover areas of community interest, including common and frequently regulated chemicals, and those relevant to high school and undergraduate chemistry classes. This chemical information, curated by our expert scientists, is provided in alignment with our mission as a division of the American Chemical Society. | |
Explanation | The data from CAS Common Chemistry is provided under a CC-BY-NC 4.0 license, unless otherwise stated. | |
Record name | 2-Iodothiophene | |
Source | ChemIDplus | |
URL | https://pubchem.ncbi.nlm.nih.gov/substance/?source=chemidplus&sourceid=0003437954 | |
Description | ChemIDplus is a free, web search system that provides access to the structure and nomenclature authority files used for the identification of chemical substances cited in National Library of Medicine (NLM) databases, including the TOXNET system. | |
Record name | 2-IODOTHIOPHENE | |
Source | DTP/NCI | |
URL | https://dtp.cancer.gov/dtpstandard/servlet/dwindex?searchtype=NSC&outputformat=html&searchlist=1082 | |
Description | The NCI Development Therapeutics Program (DTP) provides services and resources to the academic and private-sector research communities worldwide to facilitate the discovery and development of new cancer therapeutic agents. | |
Explanation | Unless otherwise indicated, all text within NCI products is free of copyright and may be reused without our permission. Credit the National Cancer Institute as the source. | |
Record name | Thiophene, 2-iodo- | |
Source | EPA Chemicals under the TSCA | |
URL | https://www.epa.gov/chemicals-under-tsca | |
Description | EPA Chemicals under the Toxic Substances Control Act (TSCA) collection contains information on chemicals and their regulations under TSCA, including non-confidential content from the TSCA Chemical Substance Inventory and Chemical Data Reporting. | |
Record name | Thiophene, 2-iodo- | |
Source | EPA DSSTox | |
URL | https://comptox.epa.gov/dashboard/DTXSID0063026 | |
Description | DSSTox provides a high quality public chemistry resource for supporting improved predictive toxicology. | |
Record name | 2-iodothiophene | |
Source | European Chemicals Agency (ECHA) | |
URL | https://echa.europa.eu/substance-information/-/substanceinfo/100.020.312 | |
Description | The European Chemicals Agency (ECHA) is an agency of the European Union which is the driving force among regulatory authorities in implementing the EU's groundbreaking chemicals legislation for the benefit of human health and the environment as well as for innovation and competitiveness. | |
Explanation | Use of the information, documents and data from the ECHA website is subject to the terms and conditions of this Legal Notice, and subject to other binding limitations provided for under applicable law, the information, documents and data made available on the ECHA website may be reproduced, distributed and/or used, totally or in part, for non-commercial purposes provided that ECHA is acknowledged as the source: "Source: European Chemicals Agency, http://echa.europa.eu/". Such acknowledgement must be included in each copy of the material. ECHA permits and encourages organisations and individuals to create links to the ECHA website under the following cumulative conditions: Links can only be made to webpages that provide a link to the Legal Notice page. | |
Record name | 2-Iodothiophene | |
Source | FDA Global Substance Registration System (GSRS) | |
URL | https://gsrs.ncats.nih.gov/ginas/app/beta/substances/Z4KJ38KVA6 | |
Description | The FDA Global Substance Registration System (GSRS) enables the efficient and accurate exchange of information on what substances are in regulated products. Instead of relying on names, which vary across regulatory domains, countries, and regions, the GSRS knowledge base makes it possible for substances to be defined by standardized, scientific descriptions. | |
Explanation | Unless otherwise noted, the contents of the FDA website (www.fda.gov), both text and graphics, are not copyrighted. They are in the public domain and may be republished, reprinted and otherwise used freely by anyone without the need to obtain permission from FDA. Credit to the U.S. Food and Drug Administration as the source is appreciated but not required. | |
Retrosynthesis Analysis
AI-Powered Synthesis Planning: Our tool employs the Template_relevance Pistachio, Template_relevance Bkms_metabolic, Template_relevance Pistachio_ringbreaker, Template_relevance Reaxys, Template_relevance Reaxys_biocatalysis model, leveraging a vast database of chemical reactions to predict feasible synthetic routes.
One-Step Synthesis Focus: Specifically designed for one-step synthesis, it provides concise and direct routes for your target compounds, streamlining the synthesis process.
Accurate Predictions: Utilizing the extensive PISTACHIO, BKMS_METABOLIC, PISTACHIO_RINGBREAKER, REAXYS, REAXYS_BIOCATALYSIS database, our tool offers high-accuracy predictions, reflecting the latest in chemical research and data.
Strategy Settings
Precursor scoring | Relevance Heuristic |
---|---|
Min. plausibility | 0.01 |
Model | Template_relevance |
Template Set | Pistachio/Bkms_metabolic/Pistachio_ringbreaker/Reaxys/Reaxys_biocatalysis |
Top-N result to add to graph | 6 |
Feasible Synthetic Routes
Disclaimer and Information on In-Vitro Research Products
Please be aware that all articles and product information presented on BenchChem are intended solely for informational purposes. The products available for purchase on BenchChem are specifically designed for in-vitro studies, which are conducted outside of living organisms. In-vitro studies, derived from the Latin term "in glass," involve experiments performed in controlled laboratory settings using cells or tissues. It is important to note that these products are not categorized as medicines or drugs, and they have not received approval from the FDA for the prevention, treatment, or cure of any medical condition, ailment, or disease. We must emphasize that any form of bodily introduction of these products into humans or animals is strictly prohibited by law. It is essential to adhere to these guidelines to ensure compliance with legal and ethical standards in research and experimentation.