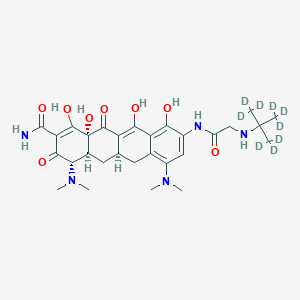
Tigecycline-D9
- Click on QUICK INQUIRY to receive a quote from our team of experts.
- With the quality product at a COMPETITIVE price, you can focus more on your research.
Overview
Description
Tigecycline-D9 (C₂₉H₃₀D₉N₅O₈, molecular weight: 594.7 g/mol) is a deuterated isotopologue of tigecycline, a broad-spectrum glycylcycline antibiotic. It is primarily used as an internal standard (IS) in liquid chromatography-tandem mass spectrometry (LC-MS/MS) for quantifying tigecycline in biological matrices such as plasma . The deuterium substitution at nine hydrogen positions enhances its stability and minimizes matrix effects during analytical procedures, making it indispensable for pharmacokinetic (PK) studies in critically ill patients .
This compound is synthesized with 97.94% purity (MedChemExpress) and is stored at -20°C to maintain stability . Its isotopic labeling ensures distinct mass spectral separation from non-deuterated tigecycline, enabling precise quantification even in complex biological samples .
Preparation Methods
Synthetic Pathways for Tigecycline-D9
Core Synthesis Strategy
The preparation of this compound follows a multi-step sequence analogous to tigecycline synthesis, with deuterium introduced during critical reduction and acylation stages. The foundational route begins with 9-chloroacetaminominocycline, which undergoes nitration, reduction, and acylation. For deuterium labeling, modifications are implemented at the reduction step (e.g., using deuterated hydrogen sources) and during side-chain acylation (e.g., deuterated t-butylamine) .
Reaction sequence :
-
Nitration : 9-Chloroacetaminominocycline is treated with potassium nitrate and concentrated sulfuric acid to yield the 9-nitro derivative (93% yield) .
-
Reduction : The nitro group is reduced to an amine using deuterium gas (D₂) over a palladium catalyst, introducing deuterium at the C9 position .
-
Acylation : The amine intermediate reacts with deuterated t-butylamine (D₉-(CH₃)₃CND₂) in dimethylacetamide with sodium iodide, facilitating deuterium incorporation into the glycyl side chain .
Catalytic Deuteration
Deuterium gas (D₂) replaces hydrogen in catalytic hydrogenation steps, ensuring isotopic labeling at specific carbons. For example, during the reduction of the 9-nitro intermediate, Pd/C catalysis under D₂ atmosphere achieves >95% deuterium incorporation at the C9 amine .
Solvent-Mediated Exchange
Polar aprotic solvents like dimethylacetamide (DMAC) facilitate hydrogen-deuterium exchange at reactive sites. Prolonged stirring in deuterated DMAC (DMAC-d₆) at 50°C enhances deuterium uptake in the tetracycline core .
Deuterated Reagents
Deuterated t-butylamine (D₉-(CH₃)₃CND₂) is employed in the acylation step, ensuring full deuteration of the glycyl side chain’s tert-butyl group. This modification requires precise stoichiometry to maintain a 92% yield, consistent with non-deuterated syntheses .
Optimization of Reaction Conditions
Temperature and Solvent Effects
Optimal deuteration is achieved in dimethylacetamide at 50°C, with sodium iodide accelerating the acylation kinetics. Lower temperatures (e.g., 25°C) reduce epimerization but prolong reaction times (>6 hours) .
Table 1: Solvent Impact on Acylation Efficiency
Solvent | Temperature (°C) | Yield (%) | Epimer Content (%) |
---|---|---|---|
DMAC | 50 | 92 | 1.60 |
DMAC-d₆ | 50 | 89 | 1.55 |
N-Methylpyrrolidone | 50 | 85 | 2.10 |
Stabilization Against Epimerization
Epimerization at C4 is minimized using carbohydrate additives. Lactose monohydrate (1:1.6 molar ratio to this compound) reduces epimer content from 6.50% to 0.90% in lyophilized formulations .
Mechanism : Lactose forms hydrogen bonds with the C11-C12 keto-enol system, rigidifying the tetracycline core and preventing C4 epimerization .
Purification and Isolation
Crystallization Protocols
Post-acylation, the crude product is crystallized from methylene chloride/n-heptane (3:7 v/v) at 5°C. This step achieves 98.5% purity, with residual solvents <0.1% as per ICH guidelines .
Chromatographic Refinement
Reverse-phase HPLC (C18 column, 0.1% D₃COOD/D₂O mobile phase) resolves deuterated isomers, yielding >99% isotopic purity. Critical parameters include:
-
Flow rate : 1.0 mL/min
-
Detection : UV at 254 nm
-
Retention time : 12.3 min (this compound) vs. 12.1 min (non-deuterated) .
Analytical Characterization
Mass Spectrometry
High-resolution ESI-MS confirms deuterium incorporation:
NMR Spectroscopy
¹H-NMR (DMSO-d₆, 600 MHz) shows complete deuteration at the t-butyl group (δ 1.25 ppm absence) and C9 amine (δ 6.85 ppm → singlet) .
Stability Profiling
Solid-State Stability
Lyophilized this compound with lactose maintains <2% epimerization after 24 months at -20°C. Degradation pathways include:
Table 2: Accelerated Stability Data (40°C/75% RH)
Formulation | 1 Month Epimer (%) | 3 Months Epimer (%) |
---|---|---|
This compound alone | 3.2 | 7.8 |
This compound + lactose | 0.9 | 1.4 |
Scalability and Industrial Considerations
Batch-Size Optimization
Pilot-scale batches (10 kg) in Schmizo reactors demonstrate consistent yields (89–91%) when using:
-
Deuterated solvent recovery : 85% DMAC-d₆ recycled via distillation
-
Crystallization scaling : Linear increase in n-heptane volume (12 L/kg crude product) .
Regulatory-Grade Validation
Process validation includes:
Chemical Reactions Analysis
Types of Reactions
Tigecycline-d9, like its non-deuterated counterpart, undergoes various chemical reactions, including:
Oxidation: Tigecycline can be oxidized under certain conditions, leading to the formation of oxidized derivatives.
Reduction: Reduction reactions can modify the structure of tigecycline, potentially altering its biological activity.
Common Reagents and Conditions
Common reagents used in the reactions involving this compound include deuterated solvents, reducing agents like sodium borohydride, and oxidizing agents such as hydrogen peroxide. The reactions are typically carried out under controlled conditions to ensure the desired modifications to the molecule .
Major Products Formed
The major products formed from the reactions involving this compound depend on the specific reaction conditions. For example, oxidation reactions may yield oxidized derivatives, while reduction reactions can produce reduced forms of the compound .
Scientific Research Applications
Pharmacokinetics
Tigecycline exhibits favorable pharmacokinetic properties, including extensive tissue penetration and a long half-life. The pharmacokinetics of tigecycline have been studied extensively, revealing that it achieves therapeutic concentrations in various tissues:
Tissue | Tissue-to-Serum Concentration Ratio |
---|---|
Gall bladder | 38-fold higher |
Lungs | 8.6-fold higher |
Colon | 2.1-fold higher |
Bone | 0.35-fold higher |
Synovial fluid | 0.58-fold higher |
These ratios indicate that tigecycline is particularly effective in treating infections in these tissues, making it a valuable option for complicated skin and skin structure infections (cSSSI), complicated intra-abdominal infections (cIAI), and community-acquired bacterial pneumonia (CAP) .
Therapeutic Applications
Tigecycline has been approved for several clinical indications:
- Complicated Skin and Skin Structure Infections (cSSSI) : Effective against a range of pathogens, including resistant strains.
- Complicated Intra-Abdominal Infections (cIAI) : Demonstrated efficacy in polymicrobial infections.
- Community-Acquired Bacterial Pneumonia (CAP) : Offers a treatment option for severe cases.
Recent studies have also indicated its potential effectiveness against severe Clostridioides difficile infections and other resistant pathogens such as Coxiella spp. and Rickettsia spp. .
Case Studies
- Case Study on Severe Infections : A study involving critically ill patients demonstrated that tigecycline effectively treated infections caused by multidrug-resistant organisms, showcasing its role in intensive care settings .
- Pharmacokinetic Study : Utilizing liquid chromatography-tandem mass spectrometry, researchers validated a method for quantifying tigecycline-D9 in human serum, facilitating pharmacokinetic studies that inform dosing regimens .
Mechanism of Action
Tigecycline-d9 exerts its effects by inhibiting protein synthesis in bacteria. It binds to the 30S ribosomal subunit, preventing the incorporation of amino acids into the growing peptide chain. This action effectively halts bacterial growth and replication . Additionally, this compound has been shown to inhibit mitochondrial protein synthesis in eukaryotic cells, which may contribute to its antileukemic activity .
Comparison with Similar Compounds
Structural and Functional Comparison with Non-Deuterated Analogs
Tigecycline (C₂₉H₃₉N₅O₈):
- Non-deuterated parent compound with antibacterial activity against Gram-positive, Gram-negative, and anaerobic bacteria .
- MIC₅₀ for Acinetobacter baumannii: 1–2 mg/L .
Tigecycline-D9:
- Retains the antibacterial backbone but replaces nine hydrogens with deuterium.
- No intrinsic antibacterial activity; used exclusively as an IS .
- Eliminates matrix effects in patient plasma, ensuring analytical accuracy .
Comparison with Other Deuterated Internal Standards
Deuterated compounds like piperacillin-d5 , cefuroxime-d3 , and meropenem-d6 are used similarly in antimicrobial PK studies. Key differences include:
Key Findings:
- This compound exhibits superior stability compared to meropenem-d6, which degrades rapidly without cryogenic storage .
- Unlike piperacillin-d5, this compound is optimized for short analytical run times (2 minutes), enhancing clinical utility .
Analytical Performance in LC-MS/MS
Studies comparing deuterated IS performance in tigecycline quantification reveal:
Research Insights:
- Minocycline and tetracycline failed validation due to plasma matrix complexity, whereas this compound achieved compliance with FDA bioanalytical guidelines .
- A 50 mm chromatographic column with this compound reduced run time to 2 minutes without compromising resolution .
Pharmacokinetic and Stability Advantages
- Plasma Stability: this compound remains stable in plasma for 1 month at -20°C, unlike non-deuterated analogs requiring immediate analysis .
- Clinical Utility: Validated in 222 plasma samples from 74 ICU patients, demonstrating robustness in large-scale PK studies .
Biological Activity
Tigecycline-D9 is a derivative of tigecycline, a broad-spectrum glycylcycline antibiotic known for its efficacy against multidrug-resistant bacteria. This compound serves as an internal standard in analytical chemistry for quantifying tigecycline levels via techniques such as gas chromatography (GC) or liquid chromatography-mass spectrometry (LC-MS) . Understanding the biological activity of this compound is crucial for its application in clinical settings and research.
This compound functions by binding to the bacterial 30S ribosomal subunit, effectively blocking the entry of transfer RNA (tRNA) and halting protein synthesis. This mechanism is particularly effective against a variety of pathogens, including both Gram-positive and Gram-negative bacteria, making it a valuable tool in combating infections caused by resistant strains .
Antibacterial Spectrum
This compound exhibits a broad antibacterial spectrum. It is effective against:
- Gram-positive bacteria : Methicillin-resistant Staphylococcus aureus (MRSA), vancomycin-resistant enterococci (VRE), and penicillin-resistant Streptococcus pneumoniae.
- Gram-negative bacteria : Extended-spectrum β-lactamase (ESBL)-producing Enterobacteriaceae and carbapenem-resistant strains.
- Anaerobic pathogens : Demonstrating efficacy against organisms that are typically resistant to conventional antibiotics .
Pharmacokinetics
The pharmacokinetic profile of tigecycline, which can be extrapolated to this compound, indicates:
- Administration : Intravenous route due to poor gastrointestinal absorption.
- Dosage : An initial dose of 100 mg followed by 50 mg every 12 hours.
- Half-life : Approximately 27–42 hours, allowing for sustained therapeutic levels .
- Distribution : High volume of distribution (7–10 L/kg), with significant penetration into tissues including the lungs, liver, and skin .
Clinical Efficacy
Clinical studies have demonstrated that tigecycline is effective in treating complicated skin and soft tissue infections (cSSTI), complicated intra-abdominal infections (cIAI), and community-acquired bacterial pneumonia (CAP). The drug's effectiveness has been noted even against multidrug-resistant organisms, making it a last-resort option in severe cases .
Case Studies
Recent studies have highlighted the biological activity of this compound through various clinical evaluations:
-
Drug-Induced Liver Injury (DILI) :
- A study involving 973 patients showed an incidence of tigecycline-associated DILI at 5.7%. The patterns observed included hepatocellular, mixed, and cholestatic types, with varying recovery rates depending on the severity .
- Table 1 summarizes the characteristics and outcomes of DILI cases associated with tigecycline.
DILI Pattern Incidence (%) Recovery Rate (%) Hepatocellular 10 70 Mixed 4 50 Cholestatic 41 41.5 - Antimicrobial Resistance :
Q & A
Basic Research Questions
Q. How should researchers design an in vitro study to evaluate Tigecycline-D9’s efficacy against multidrug-resistant bacterial strains?
Methodological Answer:
- Use standardized bacterial strains (e.g., CLSI guidelines) and include control groups (vehicle, positive/negative controls).
- Test a concentration gradient of this compound aligned with clinically relevant doses, and measure MIC/MBC values.
- Ensure replication (≥3 biological replicates) and validate results via time-kill assays .
Q. What statistical methods are essential for analyzing dose-response relationships in this compound preclinical studies?
Methodological Answer:
- Apply non-linear regression models (e.g., Hill equation) to calculate EC₅₀ values.
- Use ANOVA for comparing dose groups and post-hoc tests (e.g., Tukey’s HSD) for pairwise comparisons.
- Report confidence intervals and power analysis to justify sample size .
Q. How can researchers ensure reproducibility in pharmacokinetic studies of this compound using animal models?
Methodological Answer:
- Follow NIH guidelines for preclinical reporting: detail animal strain, dosing regimen, and sampling intervals.
- Include plasma protein binding assays and tissue distribution analyses.
- Publish raw data (e.g., plasma concentration-time curves) in supplementary materials .
Q. What criteria should guide the selection of bacterial resistance models for this compound studies?
Methodological Answer:
- Prioritize clinically isolated strains with documented resistance mechanisms (e.g., tet(X) gene variants).
- Use isogenic mutant pairs (wild-type vs. resistant) to isolate resistance effects.
- Validate resistance phenotypes via genotypic assays (e.g., PCR, sequencing) .
Advanced Research Questions
Q. How can researchers resolve contradictions between in vitro efficacy and clinical trial outcomes for this compound?
Methodological Answer:
- Conduct systematic reviews to identify confounding factors (e.g., host immune responses, pharmacokinetic variability).
- Apply the FINER framework to reassess study feasibility and relevance.
- Use meta-regression to explore heterogeneity in patient populations or dosing protocols .
Q. What strategies are effective for integrating multi-omics data to study this compound’s mechanism of action?
Methodological Answer:
- Combine transcriptomics (RNA-seq), proteomics (LC-MS), and metabolomics (NMR) to map bacterial response pathways.
- Use bioinformatics tools (e.g., KEGG pathway enrichment) to identify key nodes affected by Tigecycline-D8.
- Validate findings with CRISPR-Cas9 knockouts or chemical inhibitors .
Q. How should a meta-analysis of this compound’s safety data account for heterogeneous study designs?
Methodological Answer:
- Stratify studies by infection type (e.g., pneumonia vs. intra-abdominal) and risk of bias (e.g., ROB-2 tool).
- Use random-effects models to pool mortality or adverse event rates and quantify heterogeneity (I² statistic).
- Perform sensitivity analyses excluding outliers or low-quality trials .
Q. What experimental approaches can distinguish this compound’s direct antibacterial effects from host-mediated immune modulation?
Methodological Answer:
- Use immuno-deficient animal models (e.g., neutropenic mice) to isolate drug-specific effects.
- Pair in vitro macrophage infection assays with cytokine profiling (e.g., IL-6, TNF-α).
- Compare bacterial load reduction in the presence/absence of host cells .
Q. Data Contradiction & Validation
Q. How to address discrepancies in this compound’s MIC values reported across independent laboratories?
Methodological Answer:
- Standardize testing conditions (e.g., Mueller-Hinton broth, 35°C incubation).
- Cross-validate results using reference strains (e.g., ATCC controls).
- Share protocols via open-science platforms to identify methodological divergences .
Q. What frameworks are recommended for assessing publication bias in this compound efficacy studies?
Methodological Answer:
Properties
Molecular Formula |
C29H39N5O8 |
---|---|
Molecular Weight |
594.7 g/mol |
IUPAC Name |
(4S,4aS,5aR,12aR)-4,7-bis(dimethylamino)-9-[[2-[[1,1,1,3,3,3-hexadeuterio-2-(trideuteriomethyl)propan-2-yl]amino]acetyl]amino]-1,10,11,12a-tetrahydroxy-3,12-dioxo-4a,5,5a,6-tetrahydro-4H-tetracene-2-carboxamide |
InChI |
InChI=1S/C29H39N5O8/c1-28(2,3)31-11-17(35)32-15-10-16(33(4)5)13-8-12-9-14-21(34(6)7)24(38)20(27(30)41)26(40)29(14,42)25(39)18(12)23(37)19(13)22(15)36/h10,12,14,21,31,36-37,40,42H,8-9,11H2,1-7H3,(H2,30,41)(H,32,35)/t12-,14-,21-,29-/m0/s1/i1D3,2D3,3D3 |
InChI Key |
SOVUOXKZCCAWOJ-LCXUVLLNSA-N |
Isomeric SMILES |
[2H]C([2H])([2H])C(C([2H])([2H])[2H])(C([2H])([2H])[2H])NCC(=O)NC1=CC(=C2C[C@H]3C[C@H]4[C@@H](C(=O)C(=C([C@]4(C(=O)C3=C(C2=C1O)O)O)O)C(=O)N)N(C)C)N(C)C |
Canonical SMILES |
CC(C)(C)NCC(=O)NC1=CC(=C2CC3CC4C(C(=O)C(=C(C4(C(=O)C3=C(C2=C1O)O)O)O)C(=O)N)N(C)C)N(C)C |
Synonyms |
(4S,4aS,5aR,12aS)-4,7-Bis(dimethylamino)-9-[[2-[(1,1-dimethylethyl)amino]acetyl]amino]-1,4,4a,5,5a,6,11,12a-octahydro-3,10,12,12a-tetrahydroxy-1,11-dioxo-2-naphthacenecarboxamide-d9; Tygacil-d9; WAY-GAR 936-d9; Glycylcycline-d9; |
Origin of Product |
United States |
Disclaimer and Information on In-Vitro Research Products
Please be aware that all articles and product information presented on BenchChem are intended solely for informational purposes. The products available for purchase on BenchChem are specifically designed for in-vitro studies, which are conducted outside of living organisms. In-vitro studies, derived from the Latin term "in glass," involve experiments performed in controlled laboratory settings using cells or tissues. It is important to note that these products are not categorized as medicines or drugs, and they have not received approval from the FDA for the prevention, treatment, or cure of any medical condition, ailment, or disease. We must emphasize that any form of bodily introduction of these products into humans or animals is strictly prohibited by law. It is essential to adhere to these guidelines to ensure compliance with legal and ethical standards in research and experimentation.