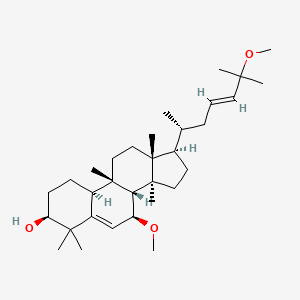
Karavilagenin A
Overview
Description
Karavilagenin A is a natural compound isolated from the herbs of Mormodica charantia L . It is a potent chemical compound with diverse applications in scientific research. Its unique structure and properties make it an ideal candidate for drug development, catalysis, and material science.
Synthesis Analysis
The synthesis of Karavilagenin A has been studied in the context of bioactive compounds of Momordica charantia . The research used in silico methods to investigate the inhibitory potential of Momordica charantia’s bioactive compounds .Molecular Structure Analysis
Karavilagenin A contains total 92 bond(s); 38 non-H bond(s), 2 multiple bond(s), 6 rotatable bond(s), 2 double bond(s), 1 five-membered ring(s), 3 six-membered ring(s), 1 nine-membered ring(s), 2 ten-membered ring(s), 1 hydroxyl group(s), 1 secondary alcohol(s), and 2 ether(s) .Physical And Chemical Properties Analysis
Karavilagenin A is a powder with a molecular formula of C32H54O3 . It is soluble in Chloroform, Dichloromethane, Ethyl Acetate, DMSO, Acetone, etc .Scientific Research Applications
Phytochemical Constituent
Karavilagenin A is one of the main constituents isolated from the species Momordica balsamina . This species, also known as balsam apple, southern balsam pear or African pumpkin, is a vegetable with high nutritional value and has been used in traditional medicine to treat several diseases .
Anti-Cancer Applications
Karavilagenin A, along with other compounds isolated from M. balsamina, have demonstrated interactions with the anti-cancer drug doxorubicin . This suggests potential applications in cancer treatment, particularly in enhancing the efficacy of existing anti-cancer drugs .
Multidrug Resistance Reversal
The cucurbitacins obtained from M. balsamina, which include Karavilagenin A, have shown selective antiproliferative activity against multidrug resistant cancer cells . They have also demonstrated their ability as P-glycoprotein inhibitors in cancer cells overexpressing this ABC transporter .
Antibacterial Applications
Karavilagenin A, as part of the cucurbitacins from M. balsamina, has been found to act as efflux pump inhibitors in resistant bacteria strains . This suggests potential applications in combating bacterial infections, particularly those resistant to common antibiotics .
Antimalarial Applications
The in vitro antimalarial activity of cucurbitacins and acyl derivatives, including Karavilagenin A, against the blood and liver-stages of Plasmodium strains has been reviewed . This suggests potential applications in the treatment and prevention of malaria .
Anti-Diabetic Effects
While specific studies on Karavilagenin A are limited, it’s worth noting that the Momordica genus, which includes the species M. balsamina, is known for its anti-diabetic effects . The anti-diabetic effects can be attributed to different bioactive substances such as vicine, charantin, glycosides, karavilosides, polypeptide-p, and plant insulin . Therefore, it’s possible that Karavilagenin A may also contribute to these anti-diabetic properties .
Mechanism of Action
Target of Action
Karavilagenin A is a natural product of the plant Momordica, specifically from the Cucurbitaceae family .
Mode of Action
It’s suggested that compounds from the same family have the ability to inhibit p-glycoprotein in cancer cells overexpressing this abc transporter, as well as efflux pump inhibitors in resistant bacteria strains .
Biochemical Pathways
Karavilagenin A, like other bioactive compounds from the Momordica charantia plant, may be involved in the Keap1-Nrf2 pathway . This pathway is crucial for cellular antioxidant responses to both endogenous and exogenous stress incidences mediated by Reactive Oxygen Species (ROS) and electrophiles .
Result of Action
It’s known that compounds from the same family have shown selective antiproliferative activity against multidrug-resistant cancer cells . Moreover, they have demonstrated in vitro antimalarial activity against the blood and liver stages of Plasmodium strains .
Action Environment
The action environment of Karavilagenin A is likely to be influenced by various factors. For instance, the plant Momordica charantia, from which Karavilagenin A is derived, is predominantly cultivated in tropical and subtropical regions . Environmental factors such as temperature, humidity, and soil composition could potentially influence the compound’s action, efficacy, and stability.
properties
IUPAC Name |
(3S,7S,8R,9S,10S,13R,14S,17R)-7-methoxy-17-[(E,2R)-6-methoxy-6-methylhept-4-en-2-yl]-4,4,9,13,14-pentamethyl-2,3,7,8,10,11,12,15,16,17-decahydro-1H-cyclopenta[a]phenanthren-3-ol | |
---|---|---|
Source | PubChem | |
URL | https://pubchem.ncbi.nlm.nih.gov | |
Description | Data deposited in or computed by PubChem | |
InChI |
InChI=1S/C32H54O3/c1-21(12-11-16-28(2,3)35-10)22-15-17-32(8)27-25(34-9)20-24-23(13-14-26(33)29(24,4)5)30(27,6)18-19-31(22,32)7/h11,16,20-23,25-27,33H,12-15,17-19H2,1-10H3/b16-11+/t21-,22-,23-,25+,26+,27-,30+,31-,32+/m1/s1 | |
Source | PubChem | |
URL | https://pubchem.ncbi.nlm.nih.gov | |
Description | Data deposited in or computed by PubChem | |
InChI Key |
OJXKMMLNVLFMJM-QTACYZFBSA-N | |
Source | PubChem | |
URL | https://pubchem.ncbi.nlm.nih.gov | |
Description | Data deposited in or computed by PubChem | |
Canonical SMILES |
CC(CC=CC(C)(C)OC)C1CCC2(C1(CCC3(C2C(C=C4C3CCC(C4(C)C)O)OC)C)C)C | |
Source | PubChem | |
URL | https://pubchem.ncbi.nlm.nih.gov | |
Description | Data deposited in or computed by PubChem | |
Isomeric SMILES |
C[C@H](C/C=C/C(C)(C)OC)[C@H]1CC[C@@]2([C@@]1(CC[C@@]3([C@H]2[C@H](C=C4[C@H]3CC[C@@H](C4(C)C)O)OC)C)C)C | |
Source | PubChem | |
URL | https://pubchem.ncbi.nlm.nih.gov | |
Description | Data deposited in or computed by PubChem | |
Molecular Formula |
C32H54O3 | |
Source | PubChem | |
URL | https://pubchem.ncbi.nlm.nih.gov | |
Description | Data deposited in or computed by PubChem | |
Molecular Weight |
486.8 g/mol | |
Source | PubChem | |
URL | https://pubchem.ncbi.nlm.nih.gov | |
Description | Data deposited in or computed by PubChem | |
Product Name |
Karavilagenin A |
Retrosynthesis Analysis
AI-Powered Synthesis Planning: Our tool employs the Template_relevance Pistachio, Template_relevance Bkms_metabolic, Template_relevance Pistachio_ringbreaker, Template_relevance Reaxys, Template_relevance Reaxys_biocatalysis model, leveraging a vast database of chemical reactions to predict feasible synthetic routes.
One-Step Synthesis Focus: Specifically designed for one-step synthesis, it provides concise and direct routes for your target compounds, streamlining the synthesis process.
Accurate Predictions: Utilizing the extensive PISTACHIO, BKMS_METABOLIC, PISTACHIO_RINGBREAKER, REAXYS, REAXYS_BIOCATALYSIS database, our tool offers high-accuracy predictions, reflecting the latest in chemical research and data.
Strategy Settings
Precursor scoring | Relevance Heuristic |
---|---|
Min. plausibility | 0.01 |
Model | Template_relevance |
Template Set | Pistachio/Bkms_metabolic/Pistachio_ringbreaker/Reaxys/Reaxys_biocatalysis |
Top-N result to add to graph | 6 |
Feasible Synthetic Routes
Q & A
Q1: What is Karavilagenin A and where is it found?
A1: Karavilagenin A is a cucurbitane-type triterpene first isolated from the dried fruit of the Sri Lankan plant Momordica charantia L., also known as bitter melon. []
Q2: What is the Structure-Activity Relationship (SAR) information available for Karavilagenin A and related compounds?
A4: The provided excerpts highlight some SAR trends observed for cucurbitane-type triterpenoids like Karavilagenins. For instance, in the study on schistosomicidal activity, the presence of free hydroxyl groups in the tetracyclic skeleton appeared crucial for activity, as compounds with sugar moieties or acylated derivatives showed reduced or no effect. [] This suggests that specific structural features within this class of compounds significantly influence their biological activity.
Q3: What are the known analytical methods for characterizing and quantifying Karavilagenin A?
A5: The provided research highlights that common techniques like Nuclear Magnetic Resonance (NMR) spectroscopy and Mass Spectrometry (MS) were crucial in elucidating the structure of Karavilagenin A and related compounds. [] These methods provide information about the connectivity of atoms and molecular weight, respectively. Additionally, techniques like column chromatography, using silica gel and Sephadex LH-20, played a role in isolating and purifying Karavilagenin A from the plant extract. []
Disclaimer and Information on In-Vitro Research Products
Please be aware that all articles and product information presented on BenchChem are intended solely for informational purposes. The products available for purchase on BenchChem are specifically designed for in-vitro studies, which are conducted outside of living organisms. In-vitro studies, derived from the Latin term "in glass," involve experiments performed in controlled laboratory settings using cells or tissues. It is important to note that these products are not categorized as medicines or drugs, and they have not received approval from the FDA for the prevention, treatment, or cure of any medical condition, ailment, or disease. We must emphasize that any form of bodily introduction of these products into humans or animals is strictly prohibited by law. It is essential to adhere to these guidelines to ensure compliance with legal and ethical standards in research and experimentation.